Three Important Design Aspects You Must Get Right Before Designing a Converter
When helping clients in the automotive industry with their DC-DC converters to meet the stringent automotive EMC standards, I often find problems that share some common mistakes. For a start, 90% of the EMI issues are associated with a ‘grounding’ issue. Poor DC link design contributes to poor conducted and radiated emissions. Another commonly seen mistake is a bad choice of switching devices, which often requires adding more filters later in the design process, significantly increasing costs and potentially jeopardizing the project.
When it comes to designing an electromagnetic (EM) compliant product, some planning is crucial to help ensure product performance and keep costs under control. In this article, we offer three recommendations addressing design aspects of DC-DC converters that will make a huge difference in their EMC performance.
The Role of DC-DC Converters in Electric Vehicles
If powertrain modules determine the performance of an electric vehicle (EV) [1], DC-DC converters then play an important role in the stability and reliability of an EV. Their primary function is to transfer energy from a source (e.g., battery pack) to systems and devices that consume that energy (all electronics loads, particularly in the low voltage distribution network of a vehicle). Because of this, the safety and functional safety aspects of a DC-DC converter are also critical. Redundancy design can also be seen for fault tolerance purposes.
There are generally two types of DC-DC converters for automotive applications. As shown in Figure 1(a), for a pure battery electric vehicle (BEV), a DC-DC converter replaces the alternator typically used in an internal combustion engine (ICE) vehicle. It steps down high voltage (HV) to low voltage (LV). The power rating of such converters is often around 3 kW.
For a hybrid vehicle or a plug-in hybrid vehicle (PHEV), a smaller power DC-DC converter (often below 1 kW) transfers power between the 48V and 12V power rails. This type of converter is often bi-directional, enabling power to go in both directions. A 48V power rail is needed for functions such as power steering, power braking, jump start, etc. Often on the 48V power rail, supercapacitors can be used to provide extra power for a very short period. This can be seen in Figure 1(b).
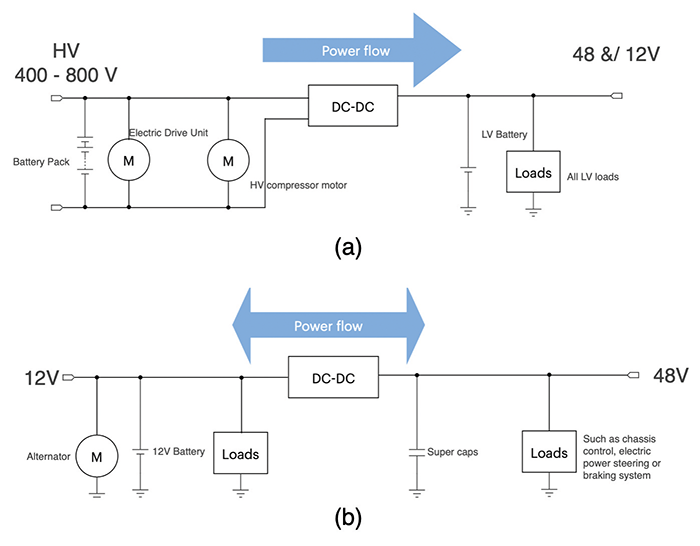
Due to the nature of high voltages and their associated safety requirements, isolation is required for HV-LV DC-DC converters. Such converters are often used with transformers since the transformer provides the isolation required. Popular topologies include phase-shift full-bridge converters and LLC resonant converters. For 48V-12V DC-DC converters, often due to the cost and size requirements, simple converter topology such as a bi-directional buck-boost converter is a popular option. In order to share the power of components, interleaving is commonly seen for both types of converters. Schematics of a phase-shift full-bridge converter and a bi-directional buck-boost converter can be seen in Figure 2.
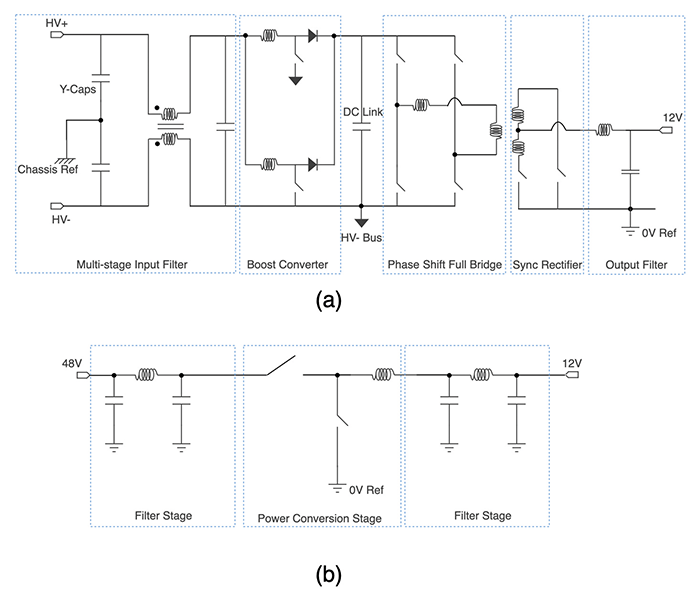
The EMC challenges associated with high voltage, high switching frequency, and fast rise time are introduced in [1]. An EMC design guide for automotive DC-DC converters can be found in [2]. In this article, we will look at the three most important design aspects that design engineers must get right in the first place to avoid the financial impact of multiple iterations of the design.
‘Ground’ Planning – First Step of a Good EMC Design
For years, EMC experts have been emphasizing the importance of having one ‘ground’ [3]. D. Beeker jokingly said that “when an EMC expert sees schematics of more than one ground symbol, he/she knows that there’s money to make.” [4]. Still, in the field, we have seen so many designs that have multiple ground symbols. They are sometimes wrongly connected or not connected to anything (as an islanded plane). But most of the time, they have connections to a reference point through a very high impedance path. Statistics drawn from our own research suggest that over 90% of the EMI issues in the automotive world are related to bad grounding design [5].
Here is a list of some commonly seen ground-related issues, which sometimes have been wrongly referred to as ‘black magic.’
- When adding more capacitors between the chassis reference and the 0V reference, the conducted/radiated emissions become worse;
- The converter is subject to transient immunity test, including ESD; and/or
- The converter is subject to bulk current injection (BCI) failure.
There is no such thing as a ‘clean’ or ‘quiet’ ground. But there’s an RF reference where the EMC measurement is taken. For an automotive DC-DC converter, it’s the chassis reference. Ideally, the filters (input and output) need to be designed using this reference. However, this cannot be achieved for many reasons, such as galvanic isolation, leakage current, and other requirements from the OEM, etc. Therefore, connections to this reference will need to be a low impedance path for the EM noise of concern.
To avoid confusion, use of terms such as ‘ground’ or ‘earth’ is not encouraged for design. Rather, we use 0V reference (or low voltage return), chassis reference, HV (–) to name our design reference. Apart from a solid continuous ground plane for the 0V reference, using a chassis reference on the PCB is a good practice. However, the chassis reference needs to be a solid continuous plane rather than a thin trace labelled as ‘chassis.’
In Figure 3, a ceramic capacitor is connected between a 0V reference (or what we call low voltage power return) and a thin trace on the PCB called ‘chassis,’ that same trace runs to a mounting hole that is connected to the metal enclosure via a stand. The problem of this is that long connections increase the current loop area; hence the inductive part of the connection begins to dominate as the frequency increases [6]. What seems to be a low impedance path becomes a high impedance path, potentially occurring at exactly the frequency point where one would like to achieve good attenuation, thereby defeating the purpose of the common-mode capacitors.
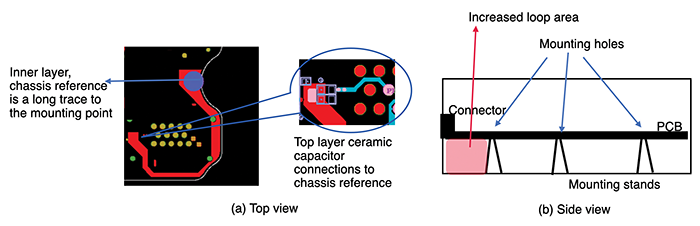
Using a large number of filter components to achieve EMC compliance is never a good option. For one
thing, it increases the size and cost of the system. It could also increase the instability due to an undamped filter. If the 0V reference and/or the chassis reference are not designed properly, adding filters can also cause an imbalance in a transmission line, particularly when a ferrite bead is used to join two reference planes. For a compact design, a quiet zone for reference plane (such as 0V reference) is often required, which means placing the filters and connectors on the opposite side of the reference plane.
If a perimeter fencing technique is used on the PCB (as shown in Figure 4), the perimeter will then need to have a good electrical connection to the metal enclosure as well. A preferred approach here is to use an EMC gasket and press it against a high-conductivity surface treatment or plating that is galvanically compatible with the EMC gasket material. Clamp the top and bottom chassis traces all around the edge of the board and between two continuous metal walls – one on the top, one on the bottom – a so-called ‘clamshell’ type of construction. This approach benefits significantly from having EMC gaskets on the top and bottom of the board. Otherwise, the EMC performance won’t last very long in real life [7].
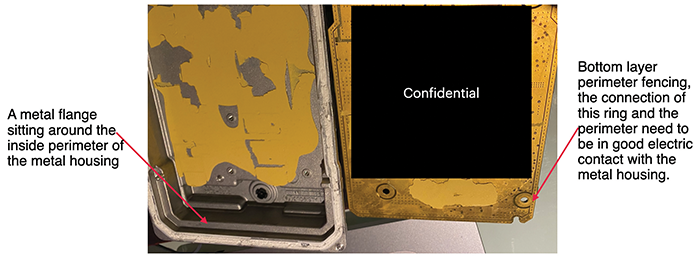
Ground planning needs to be done at the very early stage of research and development. The number of mounting points not only depends on mechanical requirements but also on EMC requirements. Once a mechanical design is fixed, it cannot be easily changed it later in the development stage because of the high cost associated with the tooling fees.
DC Link Capacitors – More Important Than You Think
Compared with applications such as grid-tied inverters of motor drives and cascaded mains power supplies like boost-LLC converters, the DC link capacitor of a DC-DC converter doesn’t need to have much energy density because power is drawn from a much higher energy density source, i.e., the battery. This explains why electrolytic capacitors are not commonly seen in such applications. DC-link capacitors for DC-DC converters consist of film and ceramic capacitors due to their low ESR and fast energy response characteristics.
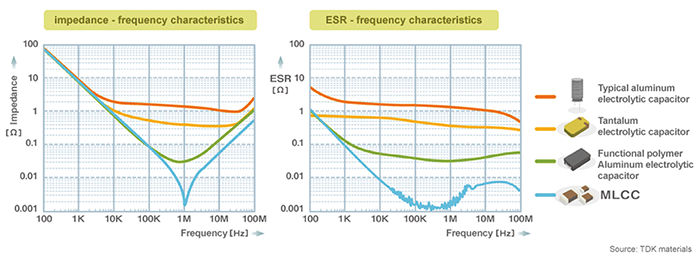
The design of a DC link requires careful system analysis, and many factors need to be considered. From the EMC point of view, voltage and current ripples on the DC link are key indicators of how good the design is.
The following process is often recommended when it comes to the DC link design, and a SPICE-based simulation model is often built to assist the analysis.
- Calculate/simulate the input current under a worst-case load scenario (duty ratio and load impedance dependent) of a DC-DC converter. For this exercise, the voltage source can be treated as an ideal voltage source, i.e., with zero impedance for simplicity.
- Calculate the source impedance. For a DC-DC converter, this means the battery internal impedance model needs to be obtained. If it is an HV battery pack, then it is the battery pack (number of cells together with their internal connections) impedance that matters.
- Cable impedance caused by both the cable itself and the wiring harness needs to be correctly modeled [8]. The important factors here are the coupling factor Leff, which is an estimated effective loop inductance per meter and the mutual capacitance between the cables. A simulated result of cable impedance can be seen in Figure 6.
- Once the source impedance and the cable impedance model are obtained, the input current harmonics can then be assessed. The results will then decide how much capacitance and what type of capacitors are needed for the DC link.
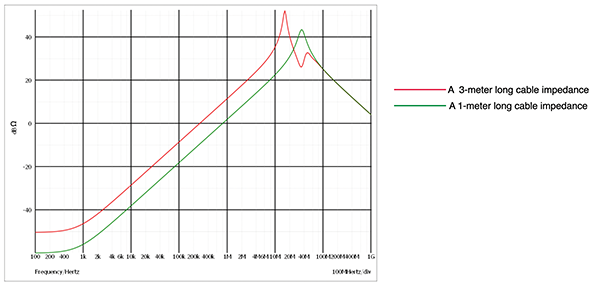
It is important to emphasize the necessity of correctly modeling the battery and the cable impedance. Ignoring the accuracy of the model often wrongly leads to the conclusion that a significantly large capacitance value will be needed. This leads to over-engineering and increases the cost and size of the unit. Cable impedance, or more precisely, cable inductance also affects the transient behavior of the system.
Electrolytic capacitors often have larger equivalent series inductance (ESL), which means the impedance starts increasing after 500kHz. Therefore, they are not the best candidates for high switching frequency converter applications. Another reason that electrolytic capacitors are not preferred is their current ripple handling capability. When the ripple current exceeds the capacitor limit, it can lead to temperature rise and reduce the lifetime of the electrolytic capacitors.
Therefore, the right choice is to have a mixture of film and ceramic capacitors to achieve a low impedance across a wide frequency range (10 kHz – 100 MHz). In order to prevent an undamped system from resonating, damping resistors are often required. Figure 7 shows the impedance characteristics of the DC link by simulation.
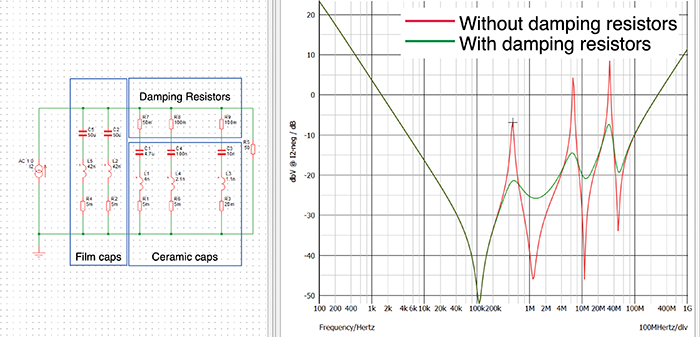
Last but not the least, the layout of capacitors needs to be carefully considered. When it comes to switched mode converters, the location of the capacitors determines how efficiently energy can be delivered from one side to the other [6]. Connections between the capacitors and the power and ground plane need to be kept short so as to minimize the inductance [6]. Depend on the converter topology, hot loop areas need to be identified and minimized to the extent possible.
Component Selection – Rethink How You Calculate the BOM Cost
For DC-DC converters, power electronics devices such as MOSFET, IGBT, and SiC MOSFET are the key components. With power electronics devices getting faster, the selection of these components becomes important [1].
Design engineers often select a device based on its voltage and current rating, RDS(ON), diode reverse recovery charge, avalanche, and thermal performance. Cost is also a key factor in making a decision since automotive manufacturing is a high-volume manufacturing business.
From an EMC point of view, we often focus on:
- The capacitance of the device (mainly output capacitance Coss)
- Reverse recovery charge of the body diode
- Gate drive circuit performance
- Parasitic inductance caused by device package
- Temperature, deadtime impact
Capacitance of the Device
The parasitic capacitance is associated with the device package and the way the device is mounted on the PCB. Generally speaking, the parasitic capacitance due to the mounting (around 100 pF) is at least one order of magnitude less than that of the device itself (around a few nF). The capacitance of the device needs to be considered because it generates the ringing of a switching event together with the parasitic inductance.
Reverse Recovery Charge
The EMC impact of the reverse recovery charge of a body diode is discussed in detail in [9].
Gate Drive Circuit Performance
A weak gate driver circuit can affect the switching performance of a converter. The miller capacitance of a device means a gate driver circuit should be designed to quickly charge and discharge the gate to source capacitance. A weak driver circuit often slows down the switch. This is bad news for system efficiency (as the switching loss increases), but often good news for EMC (as the rise/fall time increases). However, under certain circumstances, such as utilizing zero voltage switching (ZVS) of a resonant converter, a weak gate driver circuit slows down the switching. The converter doesn’t finish switching until the voltage is too high and, as a result, the ZVS is lost. When this happens, one would expect the EMC performance becomes worse.
Parasitic Inductance Caused by Device Package
The trend from through-hole packages to low-cost surface mounted device (SMD) applications is marked by the improvement of chip technologies. “Silicon instead of heatsink” is, therefore, possible in many cases [10]. There are cases in which through-hole packages such as TO-247 devices were selected for high-voltage high-power DC-DC converters. There are three main reasons for doing so. First, a through-hole package is a lot cheaper than an SMD type. Second, it makes thermal design a lot easier, as one can put heat sink directly to the devices. Third, it frees the printed circuit board area horizontally, though it does increase the height of the board.
However, a through-hole package is certainly not good for EMC. In fact, it is extremely difficult to contain the noise that is associated with through-hole devices and design engineers often must deal with the consequences of selecting through-hole devices as the main converter switches. To start with, the long lead of the package easily introduces an extra 10 nH inductance. This parasitic inductance, together with the parasitic capacitance of the device, causes over-shoot and ringing when the device is switched at a fast speed.
To demonstrate this, a SPICE-based simulation model result, together with the measurement of a TO-247 MOSFET switching is shown in Figure 8. As can be seen in Figure 9, the over-shoot and ringing are reduced by reducing the parasitic inductance.
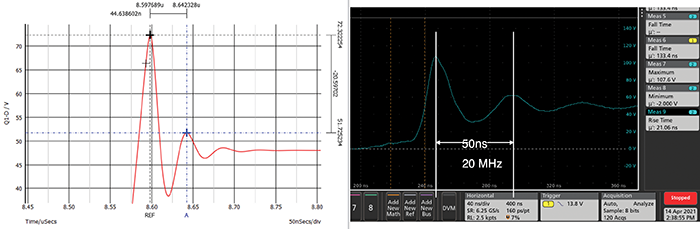
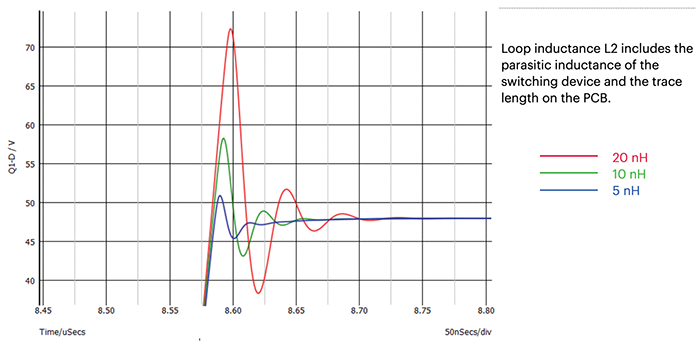
The fact that a TO-247 device can be directly mounted with a heatsink could also cause EMC problems depending on the size of the heat sink, the connections, and other factors [11]. Each device that stands on the board acts like a little antenna. If the board heat sink is not bonded correctly, each device could also increase the radiation emissions.
The layout of the devices can also pose challenges. If the through-hole devices are located near the edge of the PCB (as they often are for better thermal performance), they tend to radiate a lot more than when they are located in the middle of the PCB.
If you think selecting through-hole devices would make your bill of materials cost look better, then think again. The total number of the main power stage switching devices per DC-DC converter is not big. If an SMD package cannot achieve the same level of thermal performance compared with a through-hole package, one could always use two devices in parallel to share the current. Doubling the number of devices also means doubling the cost. But the fact that SMD packages have much better EMC performance could mean that one or two filter components can be omitted from the converter filter design.
For HV high-power converters, magnetic components such as common mode chokes (CMC) are bulky, heavy, and expensive. Adding one or two of these to the design will significantly increase the weight and the cost. To make matters worse, there is often no space to put a CMC due to the compact design of the converter. There’s no worse situation than finding yourself in a late design stage where you need to put in heavy EMI filters.
Now, knowing this, would you still choose a TO-247 package?
Temperature, Deadtime Impact
We would like to mention the impact of temperature and deadtime because we were involved in a case where significant low-frequency noise (150 kHz-10 MHz) was observed in the conducted emissions originating from a HV-LV DC-DC converter. The DC link capacitor selection and design were good where expensive high-voltage, high-volume film capacitors were selected, and the DC link bus bar meant that minimum impedance between the DC link and the switches was achieved.
But the fact that the noise was at low frequency and in differential-mode suggested that the DC link was not good enough. It turned out that the deadtime of the converter was set too small. The switching devices in this case were IGBTs and tail current was not accounted for when setting up the deadtime [12]. A short deadtime can lead to shoot through of a converter. Shoot through will cause a very high, short-duration current to be drawn from the DC link. This is most likely to happen when one of the devices has a long tail current. The tricky thing here is that tail current time is also related to temperature. In this case, the emission problem only got worse after the converter had been running for a while, which made the troubleshooting process to identify the problem a challenging task.
Conclusion
In this article, we’ve discussed three important EMC design aspects in a DC-DC converter that design engineers need to get right to avoid multiple iterations in the later stage of a design. Early ground planning, the careful choice of switching devices, and a precise DC link analysis can help ensure that your DC‑DC converter design meets the automotive EMC standards.
References
- M. Zhang, “EMC Design Techniques for Electric Vehicle Powertrain Modules,” In Compliance Magazine, February 2021.
- T. Hegarty, “An Engineer’s Guide to Low EMI in DC/DC Regulators,” 2021.
- B. R. Archambeault, PCB Design for Real-World EMI Control, Springer Science+Business Media, LLC, 2002.
- D. Beeker, “Altium Live Industry Keynote: Electromagnetic Fields for Normal Folks,” 2019.
- M. Zhang, “EMC Live Fast Circuits for Fast Cars,” 2021.
- M. Zhang, “Capacitors: Theory and Application,” In Compliance Magazine, October 2021.
- K. Armstrong, “EMC Standards – Shielding for EMC,” January 2021.
- A. Henriksson, J. Simonsson, U. Lundgren and P. Ankarson,, “Cable Modeling for Accurate Estimation of Current and Voltage Ripple in Electric Vehicles,” in IEEE Transportation Electrification Conference and Expo (ITEC), 2018.
- M. Zhang, “Demystifying EMC in an Electric Vehicle’s Drive Unit,” Interference Technology, 2020.
- R.Gulino, “Guidelines for using ST’s MOSFET SMD packages,” ST Application Note AN1703.
- T. H. Hubing, EMC question of the week 2017-2020, Learn EMC, 2021.
- I. Rectifier, “Application Note AN-983 IGBT Characteristics.”