Combination of Near- and Far-Field Measurements for Radiated Emissions
As an EMC consultant, assessing the EMC performance of large systems and machines is a common task. Over the years, I have encountered a wide range of equipment, including high-power variable-speed drives (VSDs) in factories, specialized equipment installed on ships, food processing equipment, and many others. With technological advancements, there are now even more large systems that require in-situ EMC assessment, such as quantum computers, additive manufacturing machines, waste recycling equipment, renewable energy power generators, high-power electric vehicle chargers, and more.
While testing equipment in an accredited EMC chamber is ideal, it may not be a realistic option for large machines for several reasons. First, a large chamber is required to accommodate these machines. Second, while the chamber is being charged for use, it can take days or even weeks to install the machine in a chamber and then disassemble it after the testing is complete. Finally, logistics and lead time for using the chamber can also add to the overall cost and time required for EMC testing of large machines.
Fortunately, the Technical Construction File (TCF) route to EMC compliance is available for everyone except those who manufacture radiocommunication transmitting products. Engineering companies, rather than those manufacturing mass-produced electronic products, may find the TCF route more cost-effective than the self-certification to standards route. For very large products or those that only come together on the customer’s premises, it may be impossible to test to harmonized standards anyway. In such cases, the TCF route may be the only feasible option for EMC compliance. [1]
Among the various in-situ EMC tests that manufacturers can perform, the radiated emission test is one of the most important as it demonstrates that the unit does not interfere with other equipment nearby through electromagnetic radiation. However, the radiated emissions of a large unit can be challenging to assess in situ due to two main factors.
The first factor is ambient noise, which consists of nearby radio and TV broadcast transmitters, handheld devices like walkie-talkies, equipment and machinery used during the assessment, and ESD events.
The second factor is reflections caused by metal structures, including racks, cabinets, junction boxes, conduits, and pipes. If in-situ testing is not designed and performed correctly, there can be a significant difference between chamber testing and in-situ testing, sometimes up to a 20dB difference. Therefore, it is essential to carefully consider and address these challenges during in-situ testing to ensure an accurate assessment of a unit’s radiated emissions.
The “Three-Step” Approach
In reference [2], Wyatt introduced a practical three-step approach for in-situ radiated emission assessment. The approach can be summarized as follows:
- Step 1: Conduct a near-field assessment to identify the sources of emissions, such as individual modules/components, and to determine their frequency and amplitude characteristics. This assessment often consists of two parts, one is a paper exercise, and the other is based on measurement results using near-field measurement tools;
- Step 2: Perform a cable structure radiation assessment to evaluate the emissions from cables and identify potential coupling paths; and
- Step 3: Conduct far-field measurements to assess the radiated emissions from the system as a whole.
This approach is theoretically sound and can be performed at a relatively low cost. Figure 1 lists some of the equipment that is often involved in performing both near- and far-field measurements. This article provides a detailed explanation of each step in the approach to facilitate a thorough understanding and effective implementation of the method for in-situ radiated emission assessment.
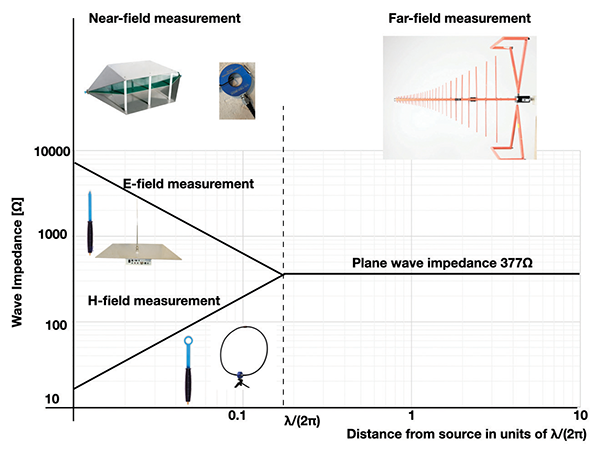
Step 1 – Near-Field Assessment
In a large unit, there can be many subsystems/modules, each with its own EMC characteristics. Some of the components are developed in-house. Therefore, engineers/system integrators will know the subsystem’s electrical and electronics architecture (EEA). From the EMC perspective, we need to know:
- The switched mode power supplies/motor drives in the subsystem, their switching frequency, and, if possible, their switching speed;
- The ICs used in the subsystem, the clock frequency, the oscillator frequency, etc.;
- Communication lines between the subsystems, whether the communication line is based on SPI, I2C, Ethernet, CAN, LIN, etc.; and
- Wireless devices, such as WiFi and BLE modules, etc.
In a large unit, it is likely that many of the modules are commercial-off-the-shelf (COTS) parts, which means that the system integrators may not have the requisite knowledge of the internal design of these devices. COTS parts may or may not come with relevant regulatory certification (e.g., CE, FCC, etc.), and it is rare for them to be accompanied by EMC test results.
It is important to note that the idea of “CE+CE=CE” is a misconception [3]. When subsystems are integrated into a single system, the EMC performance is unknown, and it cannot be assumed that the final product will automatically meet the necessary EMC requirements.
Therefore, a near-field measurement of the subsystems is essential to ensure that all clock frequencies and their harmonics are recorded, as these spectrums may appear in the far-field measurement. In cases where the subsystem/module can fit in a TEM cell, I prefer to test and record the module using the TEM cell quickly. Most of the time, the modules in a large unit may not fit in a TEM cell; therefore, we use near-field probes (both magnetic and electric field loops) to “sniff” the subsystem and record spurious levels that could potentially radiate in the far field.
It should be noted that the purpose of these measurements is not to correlate the results in the far field. Instead, the information obtained from the near-field measurements is used to determine the frequencies of critical spurious emissions in the far-field results.
Harmonics of Clock Frequencies
When documenting clock frequencies, it is important to consider their harmonics (up to the 9th harmonic). Certain harmonics can radiate more strongly than others depending on the physical structure, so it is important to take this into account during EMC testing.
A case study highlights this issue. In this example, a WiFi module from a trusted, well-established supplier was implemented in a large unit. The WiFi module passed all EMC and radio performance tests. However, the stacked board design of the unit (where the WiFi module is mounted) resulted in a structural resonance between 100 and 200 MHz. The communication between the WiFi module and the signal processing board was through the motherboard, and the clock frequency was initially set at 48MHz.
During far-field measurement, a 144MHz noise (the 3rd harmonic) was detected, exceeding the limit line. It was observed that odd harmonics of the clock frequency were radiating because of the 50% duty ratio of the clock signal. However, the data line had a broadband noise profile. When the clock frequency was reduced to 24 MHz, the 5th (120MHz) and 7th (168MHz) harmonics became high, indicating a structural resonance in the design.
This case study highlights the importance of considering the harmonics of each clock frequency during EMC testing. A noise source requires an antenna-like structure to radiate efficiently in the far field, so paying attention not only to the fundamental frequency but also its harmonics is crucial to identify potential sources of EMI and develop appropriate mitigation strategies.
Correlation Between the Near- and Far-Field Radiation
It is not recommended to use near-field measurement results to directly predict far-field emissions. This is because near-field readings are highly dependent on the geometry of the source and its properties, making it difficult to provide correlations between measurements performed in the near field and those done in the far field. While it is generally true that the stronger the field near the source, the stronger it will register in the far field, this correlation is not precise enough to provide reliable predictions [4].
IEC 61000-4-20 describes several methods for predicting radiated emissions using a transverse electromagnetic (TEM) cell, which differ in how many orientations of the device under test (DUT) are measured in the TEM cell to calculate the vector sum of emissions. The main simplifying assumption in this algorithm is that the radiating structures of the DUT have no greater gain than a dipole and a dipole radiating pattern [5]. The output of the algorithm measurements is then converted into an equivalent far-field value.
However, the effectiveness of this algorithm is limited, and a simple correlation between near-field and far-field measurements is not achievable based on tests we performed in the field. This is especially true if the module has cable connections to other modules in the system. Figure 2 demonstrates the difference between a far-field antenna measurement result and the TEM cell-predicted result.
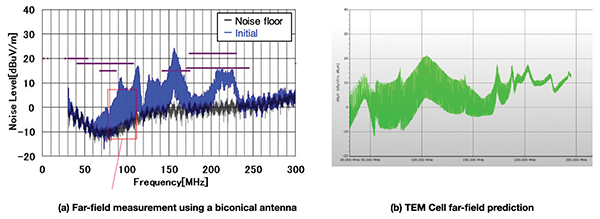
Step 2 – Measuring RF Current on Cables
After conducting a near-field assessment, the next step is to use an RF current probe to measure a sampling of cables [2]. Inside the metal chassis (this often is the cabinet that hosts the overall system), there can be hundreds of wire connections. Monitoring each individual wire or cable bundle inside the metal chassis may not be practical, so attention should be paid to cables outside the metal chassis, such as power and signal leads. A metal cabinet often serves as a Faraday cage and attenuates the field generated inside the cabinet. It is also worth “sniffing” the seam or opening of the cabinet to check for any leakage fields that could potentially radiate.
When using an RF current probe to measure cables, it is recommended to make several measurements along the cable, as standing waves on the cable can cause readings to differ between different parts of the cable. Harmonics between 30 and 500 MHz should be noted down.
While there have been discussions on predicting far-field results using current probe readings, it is often found that the cable radiation prediction method works well up to a few hundred MHz. Above 300 MHz, cables start to attenuate RF current, which may cause the prediction method to over-predict the far-field results. Readers who want to explore this topic may find References [6] and [7] useful (in which the detailed calculation method is presented), and there are software tools available that can automate the whole process and present the results once the measurement is made, as demonstrated in reference [8]. Figure 3 shows the results of the RF current prediction method against antenna measurement. Notice that the antenna measurement inevitably picks up the radio transmitter signals (in this case, both FM and DAB), while the cable prediction method does not show these ambient spectrums.
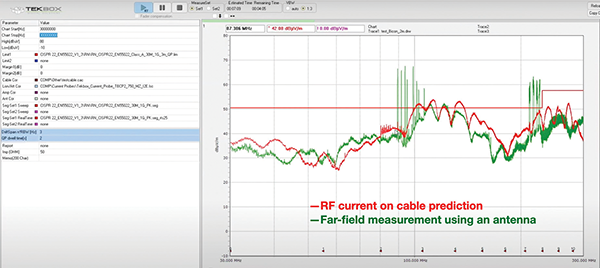
Step 3 – Far-Field Measurements
In the final step of the radiated emission assessment, the radiated emissions from the DUT are measured using antennas. Both full-size and reduced-size antennas are available in the market for this purpose. While reduced-size antennas can be advantageous for far-field measurements above 200 MHz, as they can be moved around easily and placed in locations where a full-size antenna may not fit, they may not be suitable for measuring radiation fields between 30 MHz and 200 MHz. This is because reduced-size antennas often have lower sensitivity and a higher antenna factor (AF) compared to full-size antennas, resulting in higher system noise floors that can exceed the test limits being used for comparison. Therefore, it is always recommended to use a full-size antenna for measuring radiated emissions between 30 and 200 MHz.
Reference [2] proposes a circle 3 m from the faces of the system under test, and every 30 degrees should be measured. In some cases, due to the limited space where the large unit is located, moving the antenna closer to the DUT is an option. Reducing the measurement distance from 3 m to 1 m equals approximately 10 dB less free space loss or lifting the limits 10 dB higher. However, one should consider that the antenna may move into the near-field zone at lower frequencies.
One misconception is that using an active reduced-size antenna or connecting a low noise amplifier to a passive reduced-size antenna will lower the noise floor and increase sensitivity. However, this is only true in a chamber environment where the noise floor is generally low. In a non-chamber environment, the low noise amplifier amplifies both ambient noise and the signal being measured. As a result, the spectrum analyzer will beep constantly due to RF input overloading. Therefore, reduced-size antennas are always inferior to full-size antennas in terms of performance in the lower frequency range.
When performing the far-field measurement, it is always recommended to measure the ambient noise first (i.e., while the DUT is in its off-state). For systems that cannot be easily shut down, such as quantum computers or additive manufacturing equipment, an EM survey before the system is installed is necessary, which requires early planning. Figure 4 shows an EM survey performed in a data center before the DUT was installed. One can spot the fire detection device on the wall, which radiates some narrow band spectrums. This information should be recorded in the ambient sweep.
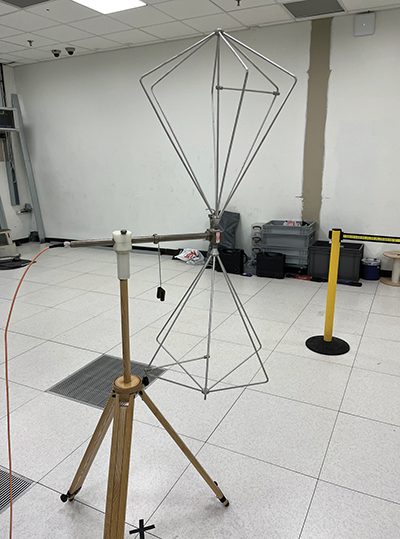
When conducting a pre-sweep of ambient noise, it is important to keep in mind that not all noise sources may be captured. Some sources may be intermittent or may only be present when other equipment nearby is turned on. Additionally, ESD events can also contribute to far-field radiation and may be picked up by the measurement antenna. In these cases, previously recorded near-field measurement results can be useful in determining whether the far-field radiation is coming from the DUT or ambient noise. Software that can load multiple results can be helpful in comparing and analyzing both the near- and far-field measurements.
An example of this is shown in Figure 5. In this case, the red trace shows the near-field measurement results while the green trace shows the far-field measurement. As it can be seen, the ambient noise can be distinguished so that we can focus on the noise generated by the DUT (the blue pointers shown in Figure 5). Quasi-peak scans can then be performed on selected points to determine whether the noise exceeds the limit line.
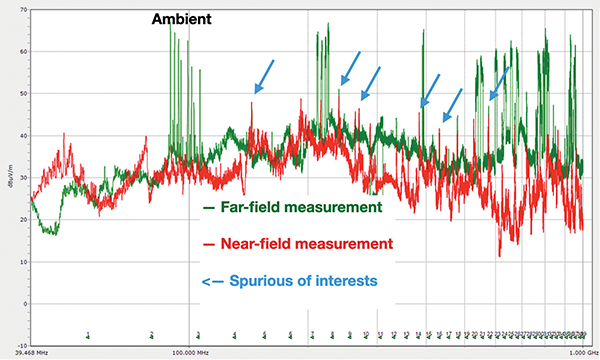
Summary
This article presents a step-by-step approach to in-situ radiated emission tests. A combined near-field, current probe, and far-field measurement are essential to get the true characteristics of the EMC performance of a large unit. Correlation methods between the near- and far-fields are discussed, and their accuracy is presented. Readers should now have a good idea of how to perform in-situ radiated emission tests.
References
- K. Armstrong, Using “Procedural” Technical Files for Compliance with the EMC Directive.
- K. Wyatt, “Pre-compliance testing large systems in-situ,” EDN.
- K. Armstrong, CE + CE does not equal CE!
- V. Kraz, “Near-Field Methods of Locating EMI Sources,” Compliance Engineering Magazine,
May/June 1995 - M. Mayerhofer, FAQ Tekbox open TEM-Cells.
- M. Aschenberg and C. Grasso, Radiation from Common Mode Currents – Beyond 1GHz (Three Methods Compared).
- A. Eadie, EMCFASTPASS, Current Probe Pre‑Compliance Testing Guide.
- M. Mayerhofer, Tekbox Application Note, Using RF Current Probe Measurements to Extrapolate to Radiated E-Field Strength.