Spectrum analyzers and scanning receivers are widely used in EMI laboratories today. Their use for measuring both narrowband and broadband signals requires specific understanding of certain instrument and signal characteristics in order to correctly interpret the displayed results. This article explains methods for the discrimination between narrowband and broadband signals and provides guidance for the proper operation of test instrumentation.
In the field of EMC, the two main categories of signals encountered are of particular importance: narrowband signals and broadband signals. The International Electrotechnical Vocabulary (IEV) defines a narrowband disturbance as “an electromagnetic disturbance, or component thereof, which has a bandwidth less than or equal to that of a particular measuring apparatus, receiver or susceptible device.” Consequently, a broadband disturbance is defined as “an electromagnetic disturbance which has a bandwidth greater than that of a particular measuring apparatus, receiver or susceptible device.” This means that the classification of a signal as narrowband or broadband is determined by the occupied frequency spectrum of the signal under investigation, relative to the resolution bandwidth (RBW) of the instrument used for measurement. If the signal spectrum is completely contained in the passband of the IF filter, it is defined as a narrowband signal. The general definition of a narrowband and broadband signal is depicted in Figure 1. It is important to note that continuous wave (CW) signals are a specific case of narrowband signals, since they consist of only one spectral line which is within the passband of the intermediate frequency (IF) filter. This case is depicted in Figure 2 (right). If the occupied signal spectrum exceeds the bandwidth of the filter, the signal is considered to be broadband. This is the case for the spectra of pulses (which are coherent signals) and noise (non-coherent signals). This scenario is shown in Figure 1 (left). This article presents various methods that are suggested for the determination of signal characteristics in EMC standards and literature. It also discusses their advantages and disadvantages. The presented material builds on previous papers that addressed the measurement of impulsive signals and discussed test equipment parameters such as the definition of impulse bandwidth and the purpose of preselection. Therefore, this article will defer to previous publications for details, as necessary.
Figure 1: Generic definition of narrowband and broadband signals
Figure 2: Two different types of narrowband signals
Narrowband and broadband signals can be generated by a variety of sources and usually represent different interference potentials for radio services. Very often an interference spectrum from equipment under test (EUT) contains both signal types. Since both signal categories require a different interpretation of the result measured with a spectrum analyzer or EMI receiver, it is essential to know the characteristics of a signal in order to correctly determine its frequency and amplitude. In some cases, the characteristics must be known in order to select the correct limit for the determination of EUT compliance. The measurement results displayed on these instruments are also dependent on some control settings, such as the sweep time and resolution bandwidth. Their impact on the measurement of signal parameters, like frequency and pulse width, must be understood to avoid erroneous interpretations of measurement results.
The Role of Instrument IF
Most modern scanning receivers, spectrum analyzers and traditional EMI receivers are super-heterodyne receivers using one or multiple stages to convert the frequency of the RF input signal to a fixed IF. This is achieved by mixing the unknown signal with a local oscillator (LO) signal in a mixing stage. Since a mixer is a non-linear device, its output includes not only the two original signals at the input but also their harmonics and the sums and differences of the input signals and their harmonics. If any of the mixed signals falls within the passband of the IF filter, it is further processed at the IF and finally displayed. After the filtering, the signal is amplified by either a logarithmic or linear amplifier, rectified by the envelope detector, possibly filtered by a low-pass filter (“Video Filter”) and finally graphically or numerically displayed.
EMI receivers as well as spectrum analyzers convert the IF signal to a video signal using an envelope detector. These signals have a frequency range from zero (dc) to some upper frequency which is determined by the detection circuit elements. In its simplest form an envelope detector consists of a diode followed by a parallel RC combination, as shown in Figure 3 (top). The output of the IF chain is applied to the detector. The time constants of the detector are chosen such that the voltage across the capacitor equals the peak value of the IF signal at all times which requires a fast charge and slow discharge time. In case the preceding resolution bandwidth of the receiver has only one spectral line in its passband (meaning, a CW signal is being measured), the IF signal is a steady sine wave with a constant peak amplitude. The output of the envelope detector will be a constant dc voltage without any variation for the detector to follow, as depicted in Figure 3 (top). However, often times there is more than one signal in the IF filter passband. For instance, in case of two sine waves, as shown in Figure 3 (bottom), these interact to create a beat note, and the envelope of the IF signal varies according to the phase change between the two sine waves. The maximum rate at which the envelope of the IF signals can change is determined by the resolution bandwidth. Since IF filters of receivers are not rectangular, the charge time of the detector needs to be a fraction of the reciprocal of the IF bandwidth (e.g. one-tenth) to obtain the envelope of the IF signal.
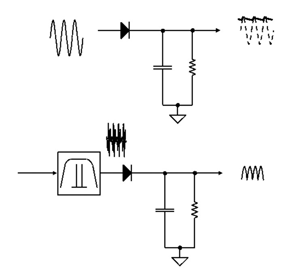
Specific instrument parameters like the selected detector, resolution bandwidth and sweep time do have an impact on the displayed measurement result, dependent on the characteristics of the signal to be measured. Therefore, they can be used to determine if a signal is broadband or narrowband.
When using spectrum analyzers or receivers for EMI troubleshooting measurements, no standard is to be applied that calls out a specific setting of the IF bandwidth. Therefore, it is mandatory to know if a measured signal is displayed as a narrowband or broadband signal in order to correctly determine the frequency of signals. Furthermore, some EMI standards like the older MIL-STD 461B provide two different limits for narrowband and broadband signals, which require a determination of the signal characteristic as part of the compliance measurement process. In both cases, suitable discrimination methods are necessary to determine a signal to be narrowband or broadband.
Resolution Bandwidth Test
As mentioned before, the reference for a signal to be broadband or narrowband is the resolution bandwidth setting of the test instrument used for the measurement. Some standards suggest the variation of the resolution bandwidth of the test instrument and observation of the resultant amplitude change of the signal under investigation. It is stated that an amplitude change, introduced by the variation of the resolution bandwidth, indicates the presence of a broadband signal. Conversely, if no amplitude change is observed, the signal is considered to be narrowband. Figure 4 depicts the measurement of an impulsive signal with a pulse repetition frequency (PRF) of 1 kHz and a pulse width of 7.7 μsec. If this signal is initially measured with a 100 Hz resolution bandwidth and the bandwidth is changed to 300 Hz, no change in amplitude is observed. Bandwidth settings that are lower than the PRF of the signal to be measured will result in the resolution of each individual spectral component. This will result in a narrowband measurement of the signal. A further increase in resolution bandwidth to 10 or 30 kHz will result in multiple spectral components located in the passband of the IF filter. A change in resolution bandwidth will result in an amplitude change of the measured signal, since wider IF bandwidths will encompass more spectral components and thus result in higher levels at the filter output. Using bandwidth settings that are wider than the PRF will indicate the presence of a broad band signal, since amplitude changes can be observed. Further increases of the resolution bandwidth to 1 MHz or greater will not yield changes in signal amplitude. This would indicate the presence of a narrowband signal, which is incorrect, in accordance with the definition. Large resolution bandwidths encompass the main spectral components of a signal (i.e., the main lobe and the first two side lobes of the spectrum), and do not lead to changes in the measured amplitude. Therefore, the variation of the resolution bandwidth as a means for determining the signal characteristic is of limited usefulness. Further information about the signal to be measured is required to avoid erroneous results. In addition, a change of bandwidth represents a change of the reference for the narrowband-broadband discrimination, which is very often neither permissible (by EMI standards) nor desirable for troubleshooting applications. It should be noted that this method provides conclusive results only when the signal under investigation is a CW signal.
Figure 4: Impact of resolution bandwidth setting on measured amplitude of broadband signal
Peak vs. Average Detection Test
A second discrimination for the determination of signal characteristics is the amplitude comparison between a peak and an average measurement. Both measurements are preferably made with the same instrument settings, especially with an identical resolution bandwidth setting. If no amplitude changes are observed between the two measurements, a signal is considered narrowband. A signal is considered broadband if an amplitude change between the two measurements is observed, with the average measurement yielding the lower amplitude. In practice, EMI standards that call out this discrimination method, like CISPR 25, specify an amplitude difference of, for example, 6 dB which is used as a decision criterion. Per CISPR 25, a signal is considered to be narrowband if the amplitude difference between the peak and average detected signal is less than 6 dB. If the amplitude difference is greater than 6 dB, the signal is determined to be broadband. This approach is meaningful since the relative amplitude accuracy of the instrument is to be considered as well as other uncertainty factors that are introduced by different instrument settings between the two measurements (e.g., change of reference level setting).
Figure 5 demonstrates the principle of this method by depicting the functionality of the peak and average detector. The peak detector will determine the envelope of the signal to be measured, which results in a low frequency signal at the detector output or a DC signal in case the signal to be measured is a CW signal. Since the peak detector determines the amplitude envelope, it will provide the maximum signal amplitudes. The average detector is often implemented as a low pass filter that is placed after the peak detector in the signal processing chain. This low pass filter, often referred to as video filter, will be used as an integrator by setting the bandwidth value to either a predefined value, called out in a standard (e.g., CISPR 16-1-1, which specifies an integration time) or to a value that is smaller than the lowest spectral component of the signal to be measured. For example, a video bandwidth setting of less than 100 Hz will result in the display of the average value of the signal depicted in Figure 4. It should be noted that the instrument is to be used in linear display mode in order to obtain the average value of the signal under investigation. The proper video bandwidth setting can be easily determined empirically by reducing the video bandwidth step-by-step and observing the resultant amplitude change. If further reductions in video bandwidth do not cause further reductions in measured amplitude, the proper video bandwidth for making an average measurement has been found.
Figure 5: Peak versus average detection
The comparison of peak and average detected signal amplitudes allows the conclusive determination of signal characteristics without changing the resolution bandwidth. This method can also be automated easily and thus allow further automation of the overall compliance measurement process.
Sweeptime Test
The presence of broadband signals is easily noticeable when a measurement is performed with a scanning receiver or spectrum analyzer. Moving responses can be observed on the instrument display; their actual location and number are dependent on the relationship of the pulse period and the sweeptime setting of the instrument. Figure 6 (top graph) shows how a scanning receiver or spectrum analyzer intercepts an impulsive signal when a slow, single sweep and peak detection is used. The impulse envelope is depicted on the vertical frequency axis, and the occurrences of the impulse are indicated by vertical frequency lines spaced along the time axis. The impulse of the period TP is detected only half way through the receiver sweep. The measured amplitude at the detection instant is determined by the envelope of the pulse spectrum, as traced out by the IF bandwidth and represents the impulse response of the receiver to the input signal. The bottom graph of Figure 6 represents the scanning receiver’s display, showing responses only at the detection instances. It is important to note that the pulse repetition frequency (PRF) cannot be determined directly from the display by measuring the frequency difference between two responses with marker functions, since a broadband signal is measured. The receiver’s IF bandwidth is much wider than the PRF; thus the displayed responses are individual input pulses separated by the pulse period and the frequency and may be calculated from the sweep time of the receiver. The correct interpretation of the measurement result is difficult without prior knowledge of the presence of a broadband signal. After a single sweep, it is not obvious that the displayed responses are due to an impulse and not caused by individual sinusoidal signals or some type of modulation. However, a narrower measurement span and longer sweep time will lead to more intercepted pulses; hence the well-recognized sin(x)/x envelope shape will be traced out, and the impulsive signal will be easily identified. Broadband signals are displayed as time domain responses with amplitudes that are proportional to the envelope of the spectrum. With the instrument tuned to a particular frequency at a point in time, the spectral lines contained within the impulse bandwidth [1] around the tuning frequency, will add periodically at a rate corresponding to the signal PRF. As the analyzer is tuned to a different frequency, the maximum pulse amplitude will change in relation to the change in the envelope of the pulse spectrum. A scanning receiver or spectrum analyzer will therefore display a response every 1/PRF seconds with an amplitude proportional to the spectrum envelope at the tuning frequency of the instrument.
Figure 6: Broadband signal detection of a scanning receiver
This phenomenon is used for the discrimination of narrowband and broadband signals. When changing the displayed frequency span on the instrument, the spacing of responses resulting from a broadband signal will not change, since they are a time phenomenon. In case of a narrowband signal, the responses are a frequency phenomenon and a change in span will cause a change in the spacing of the displayed responses. A change in sweeptime, however, will not affect the spacing of narrowband responses but have an impact on the spacing of the broadband responses. Slower sweeptimes will cause the display to show more responses, since more responses will be intercepted during a single sweep.
This discrimination method is useful to quickly determine the signal characteristic. However, if a complex spectrum is displayed, it may be difficult to observe the changes in spacing of responses.
Tuning Test
Some older commercial and military EMC standards proposed a tuning test as a method for discrimination between narrowband and broadband signals. This test involves the de-tuning of a receiver by one or two impulse bandwidths to either side of the initial tuning frequency. The initial tuning frequency is to be identical with the frequency of the maximum signal response observed. The observed amplitude change on either side is then compared to a criterion (e.g., 3 dB or 6 dB) to determine if the signal is narrowband or broadband. If the de-tuning results in an amplitude change are greater than the criterion, the signal is considered narrowband. Conversely, if the amplitude change on either side of the initial tuning frequency is less than the criterion, the signal is determined to be broadband.
This method can provide inconclusive results when the de-tuning on one side of the maximum response is larger than the criterion, and on the other side a smaller amplitude variation is determined. This situation can occur if a signal spectrum is investigated that is rather complex, which may not allow the exact determination of the frequency at which the maximum response really occurs. Furthermore, this method requires the knowledge of the impulse bandwidth of the instrument, which is not identical to the 3 dB or 6 dB bandwidth of the measuring instrument. Furthermore, this method was initially based on the use of a fixed tuned receiver, as such, this approach is not suitable for automated testing.
Summary
In the literature and standards, four main methods for the determination of signal characteristics are described. Their main aspects are summarized in Table 1.
Table 1
Their advantages and limitations have been described, and the peak versus average detector method has been identified as most suitable. This method is also called out by most EMC standards that currently require the determination of signal characteristics as part of the compliance measurement process.
Acknowledgment
The author would like to thank Mrs. Tori Barling for proof reading this manuscript.
References
- W. Schaefer, “Understanding Impulse Bandwidth Specifications of EMI receivers,” IEEE International Symposion on EMC, 1999, pp. 958-961.
- W. Schaefer, “Measurement of Impulsive Signals with a Spectrum Analyzer or EMI receivers,” IEEE International Symposion on EMC, 2005, pp. 267 – 271.
![]() |
Werner Schaefer is a compliance quality manager and technical leader for EMC and RF/uwave calibrations at Corporate Compliance Center of Cisco Systems in San Jose, CA. He has 25 years of EMC experience, including EMI test system and software design, EMI test method development and EMI standards development. He is the chairman of CISPR/A/WG1 and a member of CISPR/A/WG2 and CISPR/B/WG1. He also is the US Technical Advisor to CISPR/A and a member of ANSI C63, SC1/3/5/6/8, and serves as an A2LA and NVLAP lead assessor for EMI and wireless testing, software and protocol testing and RF/microwave calibration laboratories. He also serves as an ANSI representative to ISO CASCO, responsible for quality standards like ISO 17025 and ISO 17043. He is a member of the Board of Directors of the IEEE EMC Society. |
He was actively involved in the development of the new standard ANSI C63.10 and the latest revision of ANSI C63.4, mainly focusing on test equipment specifications, use of spectrum analyzers and site validation procedures.
Werner Schaefer is also a RAB certified quality systems lead auditor, and a NARTE certified EMC engineer.
He published over 50 papers on EMC, RF/uwave and quality assurance topics, conducted numerous trainings and workshops on these topics and co-authored a book on RF/uwave measurements in Germany.