What a Long, Strange Trip It’s Been…
Introduction1
Seventy years ago in May, the 5 microhenry line impedance stabilization network (LISN) made its debut in MIL‑I‑6181B.2 Aside from the EMI receiver itself, the LISN is one of the oldest and most successful pieces of EMI test equipment in existence. And while EMI receivers have changed a great deal since 1953 (see images in last month’s MIL‑I‑6181B anniversary article),3 the 5 uH LISN is not only still with us, but almost unchanged and used in commercial aviation and the automotive industry, as well as military applications worldwide.4 Other LISNs have come and gone, and others are with us still. The way we use LISNs has changed over time, not always for the better. But the LISN is here to stay in the world of EMI testing.
In The Beginning
Radio receivers used on WWII Army aircraft were quite susceptible to very low levels of noise on their primary (28 Vdc) power input. Further, unshielded antenna lead-ins (see Reference 3) were very susceptible to capacitive crosstalk from noisy 28 Vdc electrical power feeds.
The first EMI standards tried to control both these radio frequency interferences (RFI) coupling paths. Prior to 1953, JAN-I-2255 used a pair of 4 uF bypass capacitors in shunt (8 uF total capacity between power feeder and ground plane) and a 10’ length of power wire suspended not more than ¼” from the ground plane for what they called power supply stabilization (see Figure 1). Because these receivers tuned from 0.15 to 20 MHz, JAN-I-225 conducted and radiated emission measurements covered that same range. The resonant frequency of the 10’ wiring and 8 uF capacity occurred below the test frequency range, so that the impedance looking back into the capacitors through 10’ of wiring was inductive in character.
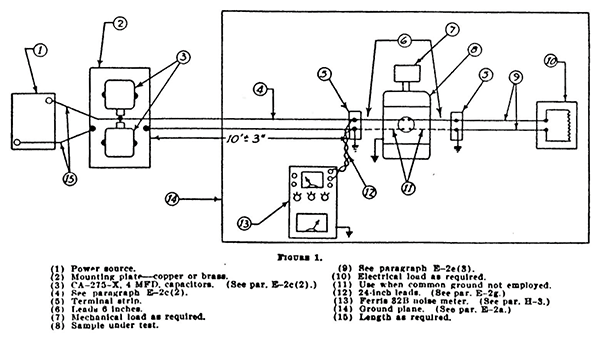
JAN-I-225 was superseded in 1953 by MIL‑I‑6181B, which included both required impedance (Figure 2) and construction drawings (Figure 3) for the 5 uH LISN. These same drawings, with two minor tweaks, appeared in RTCA/DO‑160 for commercial aircraft avionics, up to 1989.6 After that, they required the extended impedance control as in DEF STAN 59-411, but don’t include the construction details of DEF STAN 59-411. The two tweaks already appeared in MIL‑I‑6181C7 which replaced MIL‑I‑6181B in 1957: a 1 kΩ bleeder resistor from the EMI port center conductor to case and the removal of the 1 Ω resistor in series with the input side 1 uF filter capacitor.
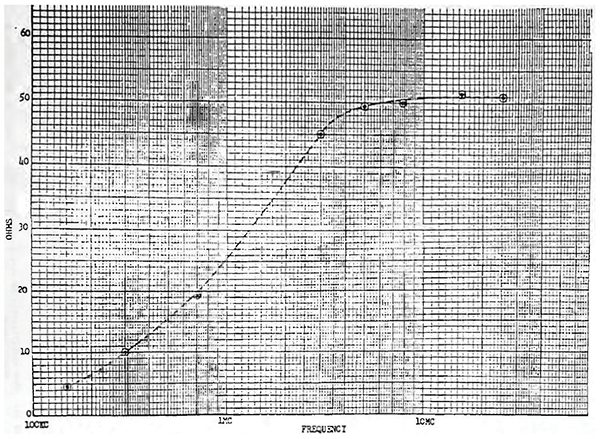
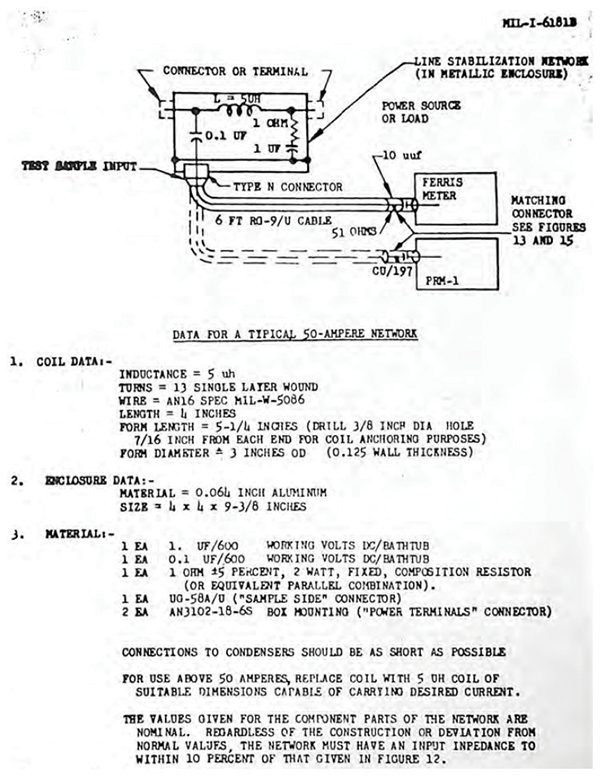
The upper frequency of the controlled impedance bounced around some over the years. MIL‑I‑6181B has it at 25 MHz, as does MIL‑I‑6181D8 (1959), but the intervening “C” in 1957 pushed it out to 100 MHz. It had settled down to 30 MHz in most specifications and standards, as that was the upper limit for conducted emissions and radiated emissions with the rod antenna. But in the past few decades, various specifications have pushed the upper end as far up as 400 MHz for rf conducted susceptibility, and the automotive world (CISPR 259) has pushed it to 100 MHz for conducted emissions.
It would surely be gratifying for the originator of the 5 uH LISN to know that his work has gained this much success and acceptance worldwide. Who was this person, and how did the 5 uH LISN come about in the first place? We are indebted to A. T. Parker (1915 – 2000), for the following historical snippet. In 1960, Parker founded Solar Electronics, a designer and supplier of EMI test equipment. Previously he had worked at the Stoddart Aircraft Radio Company, which was the company that produced the first commercial 5 uH LISN. In Parker’s own words:
“Early in WW2, an aircraft propulsion engineer named Alan Watton working for the Air Corp was concerned about the r.f., being conducted along wiring in a military aircraft of the Douglas DC-3 type. He devised the first Line Impedance Stabilization Network which simulated the impedance of the d.c. power leads in the aircraft. It used a five microhenry choke and a means for coupling voltages developed across this inductance to a 50-ohm receiver over the frequency range 150 KHz to 25 MHz.”10
This is all that Parker has to say about its inception, but there are additional facts and deductions that apply.
The DC-3 (military version C-47 “Skytrain”) was all aluminum. Aluminum aircraft return current on structure, except where inductance causes excessive voltage drop. No such problem occurs with dc power. Electrical power was from engine-mounted generators. Engine centerlines were about three meters from the aircraft centerline. Thus, using a nominal value, such as one microhenry per meter for a wire suspended above a ground plane, 5 uH seems a reasonable value if the measurement was taken in the cockpit-mounted breaker boxes, which act as the point of distribution for electrical power in the aircraft.
This point is critical. People often assume that a LISN represents the impedance the test sample sees as installed in the platform. But this is not the case.11 As shown in Figure 4, a LISN simulates the common bus impedance seen by all loads, so that noise currents drawn by a culprit load, acting through the common bus impedance, generates a noise potential inflicted on all other victim loads.
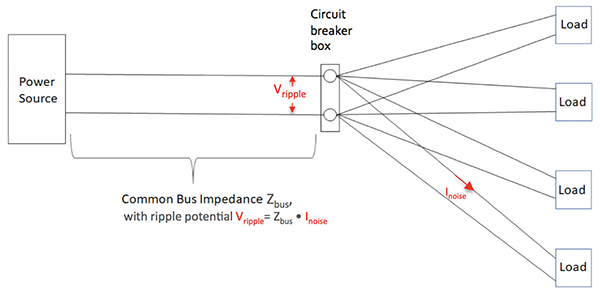
It is specifically this property of a LISN that allowed it to be used in MIL‑I‑6181B through “D” (the last revision prior to MIL‑STD‑461) in mirror image roles when measuring conducted emissions (Figure 5) and conducted susceptibility (Figure 6).
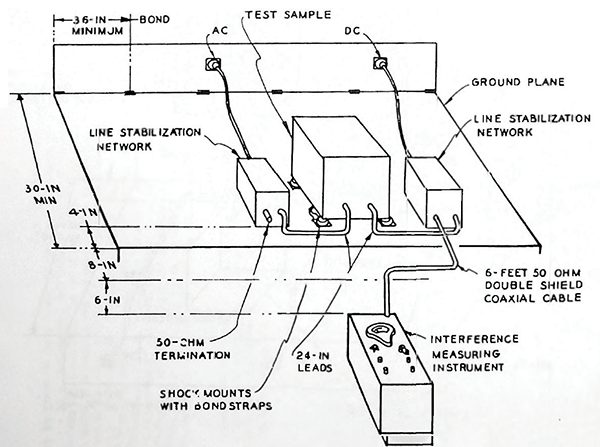
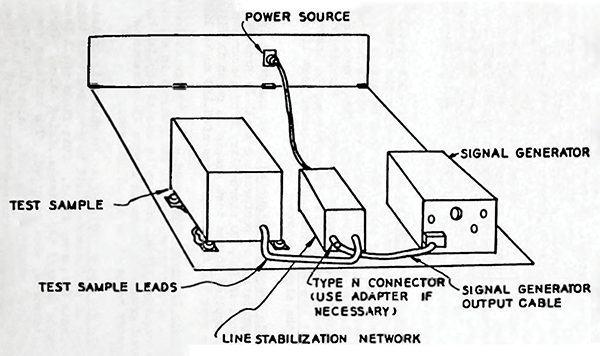
As Time Goes By
In all versions of MIL‑I‑6181B-D, a LISN is inserted in each power feeder, ac or dc. The return is always through the ground plane. But Navy ships never return current on structure, and Navy EMI specification MIL‑I‑16910A12 reflected that practice, inserting a 5 uH LISN in both feeder and return.
When all the Service- and platform-specific EMI specifications released prior to 1967 were superseded by the Tri-Service EMI standards MIL‑STD‑46113 and MIL‑STD‑462,14 it was the Navy practice of inserting line impedance stabilization in each power conductor that was adopted for Tri‑Service use. That is, instead of running return current back through the ground plane, it is returned through a wire and LISN instead.
This has several problematical consequences that reverberate down to the present day. But before delving into that issue, we should note that MIL‑STD‑461 and MIL‑STD‑462 1967 releases followed a new practice introduced in MIL‑STD‑826,15 replacing the 5 uH LISN with a 10-microfarad feed-through capacitor. This then became the standard practice for a quarter-century, until MIL‑STD‑461D16 and MIL‑STD‑462D17 reinstated rf potential instead of current control. This necessitated a LISN again, albeit now a 50 uH LISN in lieu of the original 5 uH LISN, for reasons related further on.
We return once again to Mr. Parker for the rationale behind current measurements in lieu of measuring rf potential across a LISN.18 This is follow-on to the material quoted earlier from Reference 10.
“So the Line Impedance Stabilization Network (LISN) was born. It was a pretty good simulation of that particular aircraft and the electrical systems it included. But then someone arbitrarily decided to use this artificial impedance to represent any power line.
“At any rate, this impedance suddenly began appearing in specifications which demanded its use in each ungrounded power line for determining the conducted EMI (then known as RFI) voltage generated by any kind of a gadget. The resulting test data, it was argued, allowed the government to directly compare measured RFI/EMI voltages from different test samples and different test laboratories.
“No one was concerned about the fact that filtering devised for suppressing the test sample was based on this artificial impedance in order to pass the requirements, but that the same filter might have no relation to reality when used with the test sample in its normal power line connection.
“Not until 1947, that is. At that time, this same Alan Watton, a propulsion engineer having no connection with the RFI/EMI business, decided to rectify the comedy of errors which had misapplied his original brainchild. He was in a position to place a small R and D contract with Stoddart for the development of two probes; a current measuring probe and a voltage measuring probe. Obviously, he felt that one needed to know at least two parameters for a true understanding of conducted interference…19
“As it turned out, Stoddart was successful in developing a current probe based on Alan Watton’s suggestions regarding the toroidal transformer approach which is still the primary basis used today. However, the development of the voltage measurement probe suffered for lack of sensitivity. Watton’s hope had been to provide a high impedance voltage probe with better sensitivity than was then available for measurement receivers designed for rod antennas and 50-ohm inputs. Since this effort failed and Watton’s funds (and probably his interest in the subject) faded out of the picture, the program came to a halt.
“This meant that the RFI/EMI engineer could either measure EMI voltage across an artificial impedance which varied with frequency, or he could measure EMI current flowing through a circuit of unknown r.f. impedance. Either way, the whole story is not known. In spite of the unknown impedance, the military specifications began picking up the idea of measuring EMI current instead of voltage…”
One may infer that what Watton was after was a Thévenin-like model of the test sample: “open circuit” output rf potential and short-circuit rf current. By this means, one could then predict noise potentials and currents into any arbitrary power source impedance. This interpretation is bolstered by material in the appendix of MIL‑STD‑462D:
“The (LISN) impedance is standardized to represent expected impedances in actual installations and to ensure consistent results between different test agencies. Previous versions of MIL‑STD‑462 used 10 microfarad feedthrough capacitors on the power leads. The intent of these devices was to determine the current generator portion of a Norton current source model. If the impedance of the interference source were also known, the interference potential of the source could be analytically determined for particular circumstances in the installation. A requirement was never established for measuring the impedance portion of the source model. More importantly, concerns arose over the test configuration influencing the design of power-line filtering. Optimized filters are designed based on knowledge of both source and load impedances. Significantly different filter designs will result for the 10-microfarad capacitor loading versus the impedance loading shown in Figure 7 of the main body.” (Author’s note: Figure 7 in MIL‑STD‑462D shows the impedance of the 50 uH LISN.)
The concern over designing an EMI filter for a specific (but different) source impedance is of the same type that Watton was concerned about a half-century earlier.
The more things change, the more they stay the same!
Completing our “as time goes by theme,” it is worth noting why MIL‑STD‑462D went with a 50 uH LISN instead of the 5 uH LISN. In fact, the original proposal for MIL‑STD‑462D going in was the 5 uH LISN. The same section of the MIL‑STD‑462D appendix says,
“A specific 50 microhenry LISN was selected to maintain a standardized control on the impedance as low as 10 kHz.”
The low frequency end of the 5 uH LISN is 150 kHz. The desire to begin making rf potential measurements well below 150 kHz nixed the selection of the 5 uH LISN. In turn, the reason for wanting to make rf potential measurements down to audio frequencies was based on the previous quarter-century of making CE03 measurements down to audio frequencies. They wanted the break between CE101 and CE102 to be roughly the same as between CE01 and CE03. None of which is to say that the 50 uH LISN is a better simulation of most vehicle electrical bus impedances…
Simple Things Become Complicated20
From MIL‑STD‑826 (1964) forward, the practice of placing an impedance stabilizing device in each ungrounded power lead (both feeder and return) resulted in at best questionably useful data. When a single device is used, the measured rf potential or current is simply that in the loop comprised of LISN, power feeder, load (test sample), and ground plane. Using two such devices result in measuring vector sums of differential mode (dm) and common mode (cm) currents/potentials.
Figures 7a and 7b show differential and common mode current paths when current returns above structure on a dedicated ground wire – i.e., isolated from chassis ground within the test sample. Inspection of Figures 7a and 7b indicates that, when there is an above ground current return path, differential and common mode currents sum in the feeder, but subtract in the return, as indicated in Figure 7c. Figure 7d shows how all current, regardless of the current-generating mechanism, is constrained to flow in the same path in the original structure return 5 uH LISN configuration.
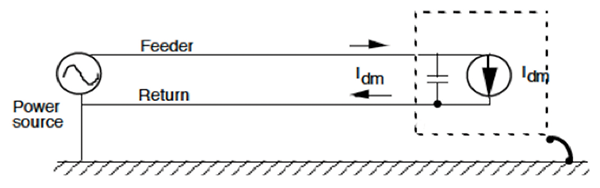
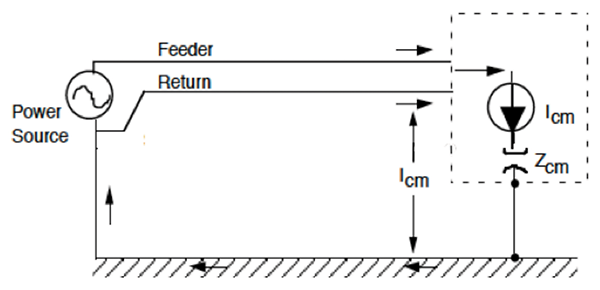
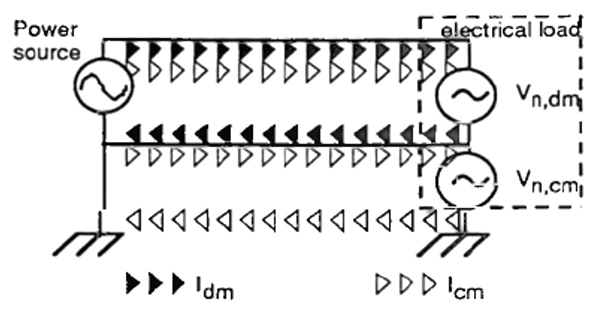
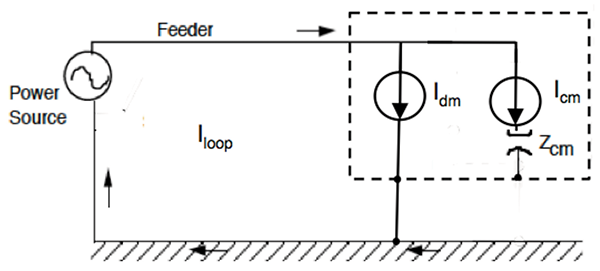
This means that with above ground current return, as shown in Figure 7c, measured single line currents or rf potentials look similar but not identical. The traces are identical for feeder and return when one or the other mode dominates, but where they are of similar amplitude and add on the feeder and subtract on the return, they differ. Separation of cm and dm modes to assist filter design has been a topic of interest since the late 1970s.21,22,23
It is of note that in most standards, if there is any question as to how power current will return (structure or dedicated wire), the default test method is to use a pair of LISNs and measure the vector sums and differences of common and differential mode signals on each LISN separately. It is not obvious why this is the go-to default. Particularly for radiated emissions, this technique decreases the radiation efficiency of the differential mode component of the composite noise (especially if, as is common, the wire pair is twisted). Figure 7d makes it clear that using a single LISN keeps the radiation efficiency of each mode identical.
When we know that current will be returned on a dedicated wire, not on structure, a better technique than controlling emissions on each individual lead is controlling emissions by mode. Separating modes may be done directly off the LISN (References 20 – 22) or using current probes. Regardless, if we control emissions via mode, not line, we can then assign limits based on what the modes actually affect:
- Differential mode noise currents cause ripple, and
- Common mode currents cause radiated emissions
Therefore, when the feeder and return wires are twisted or held tightly together throughout the vehicle, it is reasonable to relax the differential mode limit compared to the common mode limit. Even if no radios operate in the conducted emission frequency range, it may be worthwhile to control common mode emissions to limit crosstalk to adjacently placed cables that might carry potentially susceptible low level signals.24
A concrete and illuminating example of the problem of LISN misuse may be found in a report by the author dating to the late 1990s.25 This report showed that the (now obsolete) FCC Class B 48 dBuV conducted emission limit was in fact 20 dB too stringent for differential mode noise but was precisely correct for common mode noise. The problem arose because the original work done to establish the 48 dBuV limit was performed using a single 5 uH LISN, but the FCC test method was based on a pair of (50 uH) LISNs.26 It was not the disparity in the LISN impedance but the mode separation inherent in a pair of LISNs that demonstrated the disparity.
Another modern confusion is using long power leads between the LISN and test sample. Such values range from one meter (for conducted emissions) in MIL‑STD‑462 (1967 – 1993), 2 – 2.5 meters in MIL‑STD‑462D and follow-on versions of MIL‑STD‑461, one meter in RTCA/DO-160, and 1.5 meters in CISPR 25. By way of contrast, the specified length in MIL‑I‑6181B was 24 inches.
Consider the ramifications with respect to measurement uncertainty. First, MIL‑I‑6181B conducted emission limits stopped at 20 MHz. The electrical length of a 24” long wire at 20 MHz is a twenty-fifth wavelength. VSWR will be negligible, and therefore the LISN does in fact control the power source impedance seen by the test sample. MIL‑STD‑462D and follow-on MIL‑STD‑461 versions using a 2.5-meter-long power lead and 10 MHz upper CE102 limit frequency come in at less than a tenth-wavelength, so the LISN controls the power source impedance.
But look at specifications such as RTCA/DO-160 and DEF STAN 59-411, with 400 MHz LISNs and 100 MHz conducted emission control. A one-meter-long power lead is a third wavelength at 100 MHz. And for CISPR 25, using a two-meter-long power wire, the LISN is over a half-wavelength from the test sample. All the work and expense that went into the extended frequency range LISN is wasted when the parasitics controlled within the LISN is simply migrated to the LISN – test sample interconnection.27
Conclusion
Alan Watton bequeathed us a great gift some seventy years ago. It is up to us to use it wisely, and well. To echo Parker about the comedy of errors, and intentionally misquote Gall’s Law, “A complex system that works poorly is invariably found to have evolved from a simple system that worked well.”
Acknowledgments
The author wishes to thank the reviewers for their time and effort in making this article useful. Any errors of omission or commission are the author’s own.
Endnotes
- Visit http://www.emccompliance.com to find all specifications, standards, and other sundry documents cited herein that are not copyrighted by others.
- MIL-I-6181B, Interference Limits, Tests and Design Requirements, Aircraft Electrical and Electronic Equipment, 29 May 1953
- Javor, K. “Seventy Years of Electromagnetic Interference Control in Planes, Trains and Automobiles (and Ships and Spaceships, as well),” In Compliance Magazine, May 2023.
- Ministry of Defence Standard 59-411 and the older 59-41 all use a modification of the 5 uH LISN. The modification extends the frequency range of controlled impedance down to 1 kHz and up to 400 MHz. It is less than obvious why the LISN impedance needs to be controlled to 400 MHz when it is placed several meters from the test sample. The mismatch between power wire transmission line characteristic impedance and the 50 Ω LISN is always going to generate reflections, no matter how well the LISN impedance is controlled. If it is desired to have true impedance control, the LISN needs to be within a tenth-wavelength of the test sample power input. A 10 uF feedthrough capacitor would function admirably so used, at a tenth the cost of the 400 MHz LISN.
- JAN-I-225, Interference Measurement, Radio, Methods Of, 150 Kilocycles to 20 Megacycles (For Components and Complete Assemblies), 14 June 1945
- RTCA/DO-160 original through C revision: Environmental Conditions and Test Procedures for Airborne Equipment
- MIL-I-6181C, Interference Control Requirements, Aeronautical Equipment, 06 June 1957
- MIL-I-6181D, Interference Control Requirements, Aircraft Equipment, 25 November 1959
- CISPR 25 all editions, various titles. “Limits and methods of measurement of radio disturbance characteristics for the protection of receivers used on board vehicles” is the 1995 title.
- Parker, A. T. “A Brief History of EMI Specifications,” presented at the 1992 IEEE EMC Symposium. The Army Air Corp to which Parker refers was the forerunner of the US Air Force. The Army Air Corp became the United States Air Force in 1947.
- Some exceptions that prove the rule are many spacecraft line impedance simulation networks that appear to be designed to include the dedicated wiring to the test sample itself. See the line impedance simulation section of older print Solar catalogs (they no longer supply spacecraft LISNs, so the on-line catalog is of no value here). Pay special attention to the series resistance value. Values above a few tens of milliohms mean they are simulating the entire power distribution network, not the main bus. As Mr. Parker said in his catalogs, in his gentlemanly way, “Spacecraft designers do not always agree on the characteristics of the d.c. power source aboard the vehicle. The inductance in series with the load, the resistance across the inductor, and the series resistance in each leg of the unit are variables specified by different spacecraft engineers.”
- MIL-I-16910A, Interference Measurement, Radio, Methods and Limits: 14 Kilocycles to 1000 Megacycles, 30 August 1954
- MIL‑STD‑461, Electromagnetic Interference Characteristics, Requirements, Electrical for Equipment, 31 July 1967
- MIL‑STD‑462, Electromagnetic Interference Characteristics, Measurement of, 31 July 1967
- MIL-STD-826, Electromagnetic Interference Test Requirements and Test Methods, 20 January 1964
- MIL‑STD‑461D, Requirements for the Control of Electromagnetic Interference Emissions and Susceptibility, 31 January 1993
- MIL‑STD‑462D, Measurement of Electromagnetic Interference Characteristics, 31 January 1993
- Solar Electronics Application Note AN622001, “Using the Type 6220-1A Transformer for the Measurement of Low Frequency EMI Currents.” The application note used to be included in Solar Electronics catalogs. The excerpted portion is still found on their website under “Audio Isolation Transformers” under “History.”
https://www.solar-emc.com/6220-1B.html - Author’s comment about the 1947 date cited in this paragraph. 1947 seems too early. That is before MIL-I-6181, which used JAN-I-225, which didn’t include the 5 uH LISN. The date 1957 fits better, because MIL-I-6181C released in that year for the first time includes an alternate conducted emission test method and limit based on the use of a current probe, for cases when line current exceeds the 50 ampere LISN maximum current rating. But there is no way to know for certain if this was a typo, or bad memory or some other explanation.
- For much more on the topic of conducted emission mode separation, see the expanded version of this article on the author’s website, and other articles by this author and those listed as references on this topic.
- A. A. Toppeto, “Test Method to Differentiate Common Mode and Differential Mode Noise,” Proc. 3rd Symposium on Electromagnetic Compatibility, Rotterdam pp. 497-502, May 1979.
- M. J. Nave, “A Novel Differential Mode Rejection Network,” IEEE International Symposium on Electromagnetic Compatibility, Denver, May 1989.
- LISN UP Application Note, Fischer Custom Communications, 2005.
- Two spacecraft specifications follow this approach, where it is known that no current of any sort returns on structure. These spacecraft don’t operate radios in the bands where conducted emissions are controlled; the imposition of a common mode limit is based purely on controlling crosstalk. The resulting common mode limit is sufficient to the task and represents a large relaxation relative to typical radiated emission limits that protect against radio frequency interference.
GSFC-STD-7000B, General Environmental Verification Standard (GEVS) for Goddard Space Flight Center Flight Programs and Projects, 29 April 2021
GP 11461, Gateway Requirements for the Control of Electromagnetic Interference Characteristics of Subsystems and Equipment, 06 November 2019 - Javor, Ken. “Investigation Into the Susceptibility of Radio Receivers to Power-Line Conducted Noise” EMC Compliance, 1998. Technical committee presentation and demonstration at 1998 IEEE EMC Symposium, Denver
- CBEMA/ESC5/77-29, Limits and Methods of Measurement of Electromagnetic Emanations from Electronic Data Processing and Office Equipment, 20 May 1977
- MIL‑STD‑462 (1967) had a unique approach to this conundrum. It specified one-meter-long wires for conducted tests, and two-meter-long wires for radiated. The only problem with that approach was not enough people followed directions; opting for one or the other length for both conducted and radiated measurements. The end result was that when the “D” revisions came along, they “dumbed down” the standard to one length optimized for radiated and reduced the conducted frequency range accordingly. Hence the 10 MHz upper limit for conducted emission control, unique amongst conducted emission limits. The goal for standardized test results outweighed the desire to control conducted emissions out to the traditional 30 MHz.