Antennas, Patterns, and Ground Benches for Testing for Automotive Components and Full Vehicles
This two-part article is an update of the original article authored by Dr. Vince Rodriguez, then with ETS‑Lindgren. An earlier update was published in the February 2016 issue of In Compliance Magazine.
In Part 2 of this article, we shall talk a bit about the antennas used for automotive EMC testing. Specifically, we are going to concentrate on the typical biconical, LPDA, and DRH antennas recommended for CISPR 25, and the DRH antenna recommended for ISO 11452-2.
Recently it has become important to understand the radiation characteristics of these antennas. The typical biconical antenna as shown in Figure 1 is an omnidirectional radiator. Its pattern shown in Figure 2 at 100 MHz is typical of the radiation pattern across the entire range. From these patterns, we can extract the half power beam width (HPBW). For the H plane, it is clear that the HPBW is larger than 180 degrees, and there is no main beam. For the E plane, the beamwidth ranges between 40 and 90 degrees. On the measured data, we can see the effects of the stem and balun holder on the pattern. The stem is oriented to the 180-degree mark. We can see how on the H plane the balun holder reduces the intensity of the radiation by 2 to 3 dB. The beamwidth of the measured data and the computed data track each other nicely.

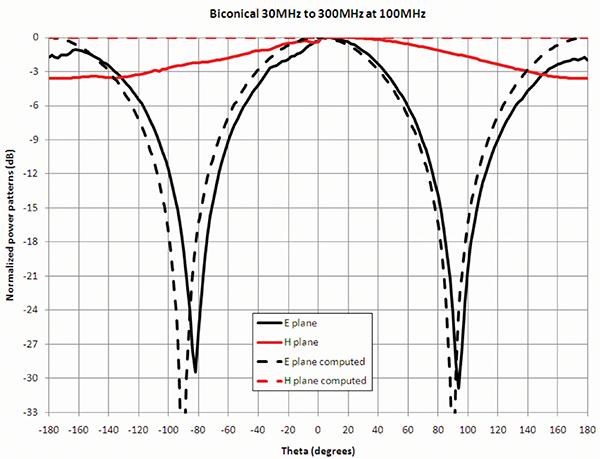
Figure 3 shows a picture of an LPDA antenna and the numerical model created with specialized software. This is the other typical antenna type recommended by CISPR.
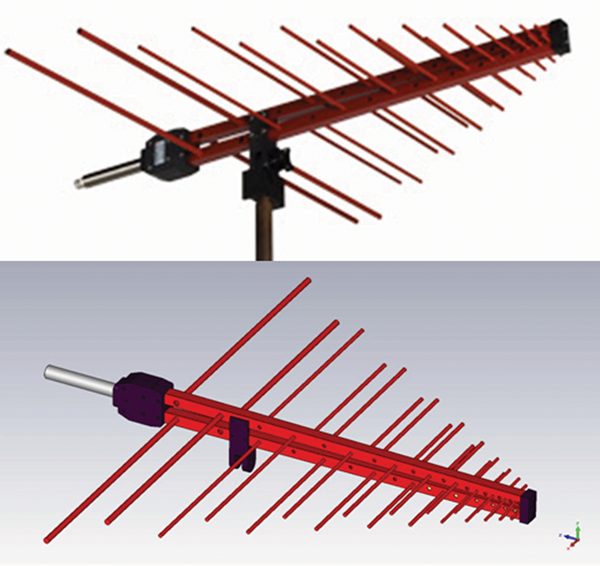
In Figures 4 and 5, we see the measured and modeled performance of the LPDA antenna. There are clearly some differences between the measured data and the computed results. Close examination reveals that the error is under 3 dB. There are several sources of error in the measurement. Using the measured values for the HPBW, the EMC engineer will err on the side of safety.
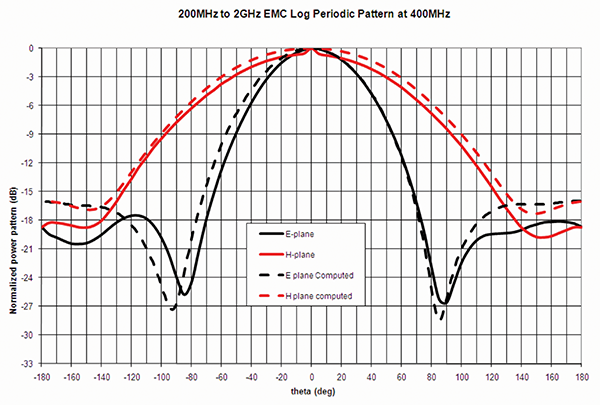
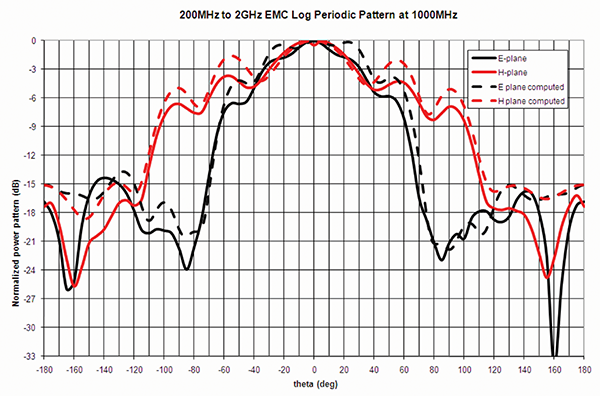
Figure 4 shows the data at 400 MHz, in which there is very good agreement between the measured and the computed results. The data for 1 GHz (shown in Figure 5) shows good agreement between measured and computed data for the main beam.
The HPBW of the LPDA antenna is usually fairly flat. This is especially the case for the center of the frequency band covered by the antenna. From about 200 to 1000 MHz, the antenna being measured exhibits an HPBW ranging from 100 to about 60 degrees for both planes.
DRH antennas are the antenna of choice for higher frequencies. This family of antennas has been described numerous times in the literature. Their radiation pattern has been widely described. Reference [6] describes issues with the radiation pattern of these antennas at frequencies above 12 GHz for models operating in the 1 to 18 GHz range. References [7] and [8] introduce a new design for the 1 to 18 GHz range that has a better-behaved pattern where the main beam does not split into multiple beams. Figure 6 shows the measured radiation patterns for the horn analyzed in [6] and the one introduced in [7] and [8]. The data on the left shows a better-behaved pattern than the antenna on the right without the narrow beams and the split main lobe of the pattern.
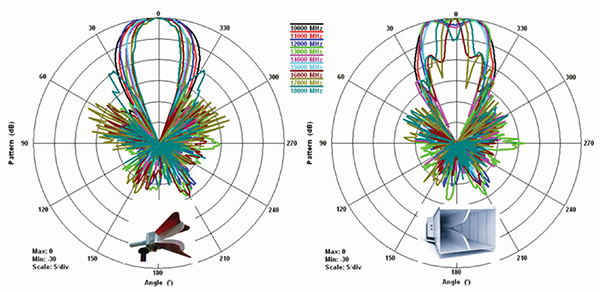
In References [8, 9] several improvements were made to the radiation patterns of DRH antennas operating in the 200 MHz to 2 GHz range. These are the horns we recommend for ISO 11452-2 since the modifications correct the nulls in the middle of the main beam.
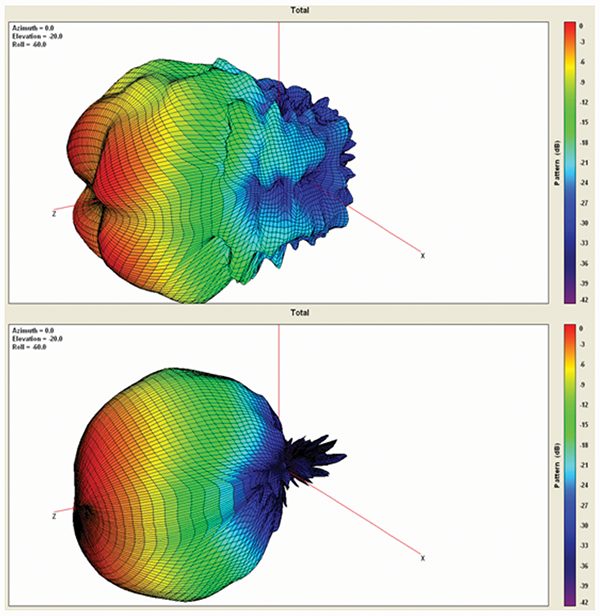
It is important to keep in mind that the data shown for the patterns is free space and far-field data. While it is true that it provides an idea of the antenna coverage, it can be misleading once we are in the presence of conductive benches. Figure 8 shows a typical setup for either CISPR 25 or ISO 11452-2. An antenna is placed 1 m away from the bench that is grounded. For the horizontal polarization case, Figure 9 shows the dramatic effect that the bench has on the fields. While the cable harness will be covered by the antenna, the EUT will barely be in the illumination. This happens at all frequencies and it is related to the boundary conditions that are part of the electromagnetic phenomena.
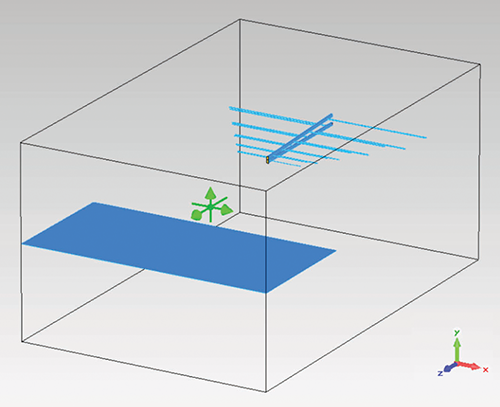
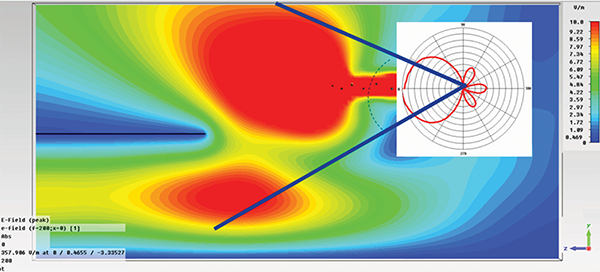
The LPDA, DRH, and SGH antennas have been a stable and long-standing part of immunity measurements for many years. Within this period we have witnessed the development of model variants with higher gain, customized bandwidths (for radar pulse testing, for example), extended bandwidths, and higher power handling, all in an effort to improve the efficiency of the measurement setup with reduced antenna changes and reduced amplifier power.
This trend is continuing, and we have already started seeing the emergence of the next generation of immunity antennas.
The DRH antenna remains an attractive antenna for automotive EMC testing largely due to its wide operating bandwidth, stable radiating characteristics, and small size. However, the lower gain at its lower frequency end drives the need for high amplifier input power, making it impractical to achieve the required high field strength as required by ISO 11542-2 in some instances. In addition to achieving higher field levels for many immunity tests, it is also critical that the field uniformity (FU) requirements are satisfied (also required by ISO 11451-2). It is accepted that higher antenna gain is typically associated with narrower beam width which may lead to FU deterioration, so finding the correct compromise of size, gain, bandwidth, and beamwidth remains one of the antenna designer’s goals.
To solve this problem, horn antennas with lenses have become increasingly popular for automotive EMC testing applications. With dielectric lenses having properties such as low loss and wide operational frequency range, ridged horn antennas have been able to meet both field strength and FU requirements for automotive EMC testing in the 1 – 5 GHz frequency range. Figure 10 shows how adding a lens to a ridged horn antenna can drastically improve the gain vs bandwidth balance.

A ridged horn antenna with a lens (1-3.1GHz), mounted over a stand, is shown in Figure 11. Its lightweight meta-material lens increases the gain of the horn at a 1 m distance by 9 dBi. This characteristic makes the antenna ideal for automotive component immunity testing. Such high-gain antennas help to meet the narrow band high field strength requirement with less input power for automotive immunity testing. Figure 12 shows the power vs frequency plots required for this antenna to achieve 200V/m and 600V/m.
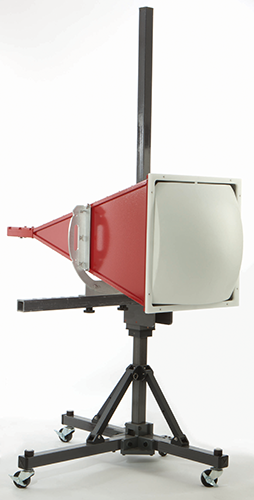
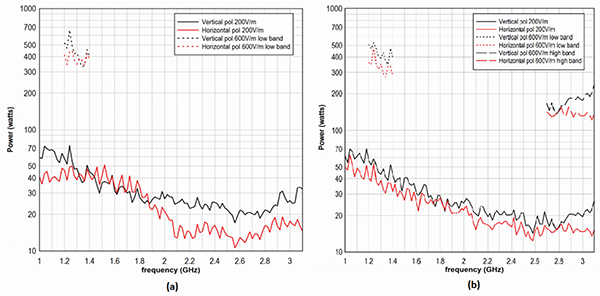
As described previously, a compliant CISPR 25 chamber with a 2m long ground plane bench for component testing can be as small as 6.2m x 5.3m x 3.6m. For full vehicle testing, however, a larger chamber is needed depending on vehicle size, test range length, and testing scope. The EMC chamber facility shown in Figure 13 is an example of a full vehicle chamber where the hybrid polystyrene absorbers previously mentioned in Part 1 of this article have been used to achieve the desired test volume reflectivity performance. The interior dimensions of this 10-meter chamber are approximately 20.8m x 12m x 8m with a 5m diameter quiet zone and 10m range according to CISPR 16‑1-4. Absorber coverage was provided on all wall and ceiling surfaces (see Figure 13). This newly retrofit chamber has been designed for automotive and commercial EMC testing in accordance with international standards CISPR 12, CISPR 25, ISO 11451, ISO 11452, and IEC 61000‑4‑3, as well as military standard MIL‑STD‑461E/F.

More recent chambers with a hybrid layout as the example shown in Figure 14 have been designed to also support antenna pattern measurements. In this example, the chamber has overall dimensions of 54m x 15m x 14m height including the 18m x 15m rectangular section. This chamber is also fully lined with the polystyrene absorber material providing optimum performance for EMC measurements with satisfactory performance for the low and intermediate frequency antenna pattern measurements. This chamber was designed to meet the CISPR 12/16/25, ISO 11451/11452, R10, SAE, and ANSI C63.4 standards.

Conclusion
In this two-part article, we have introduced the two main standards for automotive vehicles and components with an overview of the revision status of these and several related standards produced by CISPR and ISO. We have concentrated on designing a chamber to meet the requirements of CISPR 25 and showed that the same chamber is usable for ISO 11452-2. Finally, we have shown some radiation patterns of the typical antennas recommended by the standards, the performance improvements for a ridged horn fitted with a lens, and the benefits of reducing the power demand. The various patterns will give the user an idea of the illumination area that the antennas cover when used, and how the presence of the bench can have a dramatic effect on the radiation pattern and the coverage of the antennas. This is clearly an aberration caused by the setup used for these standards and not by the antennas being used. So, as with most measurements, caution is recommended in the selection of antennas, set up, and validation steps taken to verify that the intended fields are present over the entire area of the EUT to account for any distortions or resonances that may be present.
In closing, the chamber installation example we’ve presented here highlights the notion that, wherever possible, new installations should take advantage of the best available technology and the latest revisions of the relevant standards, as is shown with the use of the proposed CISPR 25 5th Edition chamber validation method and, as in the case of a hybrid design, other tests and standards can be accommodated with careful absorber selection and treatment.
Acknowledgment
The authors would like to thank Mr. Stéphane Blanc of UTAC CERAM Group for providing the measurement data of their automotive EMC 10-meter chamber, and the engineers at NISSAN for the opportunity to collaborate on a novel chamber design to support EMC as well as antenna pattern measurements.
References
- CISPR 12, Vehicles, boats and internal combustion engines – Radio disturbance characteristics – Limits and methods of measurement for the protection of off-board receivers, Sixth Edition, IEC Geneva, Switzerland, 2007.
- CISPR 25, Radio disturbance characteristics for the protection of receivers used on board vehicles, boats, and on devices- Limits and methods of measurement, Fifth Edition, 2021.
- MIL-STD 461, Requirements for the control of Electromagnetic Interference Characteristics of Subsystems and Equipment, Department of Defense Interface Standard 10, December 2007.
- ISO 11452, Road vehicles – Component test methods for electrical disturbances from narrowband radiated electromagnetic energy – Part 2: Absorber-lined shielded enclosure, Second Edition.
- CISPR 16-1-4, Specification for radio disturbance and immunity measurement apparatus and methods, Part 1-4: Radio disturbance and immunity measuring apparatus – Antennas and test sites for radiated disturbance measurements, Third Edition, IEC Geneva, Switzerland, 2010.
- C. Bruns, P. Leuchtmann, and R. Vahldieck “Analysis of a 1-18 GHz Broadband Double-Ridge Antenna,” IEEE Transactions of Electromagnetic Compatibility, Vol 45, No. 1, pp.55-60, February 2003.
- V. Rodriguez “New Broadband EMC Double-ridge Guide Horn Antenna,” RF Design, May 2004, pp. 44-50.
- V. Rodriguez, “A New Broadband Double Ridge Guide Horn with Improved Radiation Pattern for Electromagnetic Compatibility Testing,” 16th International Zurich Symposium on Electromagnetic Compatibility, Zurich, Switzerland, February 2005.
- V. Rodriguez, “Improvements to Broadband Dual Ridge Waveguide Horn Antennas,” 2009 IEEE International Symposium on Antennas and Propagation and USNC/URSI National Radio Science Meeting, Charleston, SC, June 1-5, 2009.
- V. Rodriguez, “Recent Improvements to Dual Ridge Horn Antennas: The 200 MHz to 2 GHz and 18 GHz to 40 GHz Models,” 2009 IEEE International Symposium on EMC, Austin, TX, Aug 17-21, 2009.
- CISPR 36, Electric and Hybrid Electric Road Vehicles – Radio disturbance characteristics – Limits and methods of measurement for the protection of off-board receivers below 30 MHz, First Edition, IEC Geneva, Switzerland 2020.
A Most Memorable Meeting of the ISO/CISPR D Automotive EMC Committee
by Janet O’Neil
The most recent meeting of the ISO and CISPR D Automotive EMC Committees was held in October 2022 at ETS-Lindgren in Cedar Park, Texas. Over a nearly two-week period, more than 60 global automotive experts gathered to address the latest automotive EMC measurement techniques in the CISPR 12, CISPR 25, CISPR 36, ISO 11451-x, and ISO 11452-x standards.
|
|
Behind the scenes, committee members were also planning to honor Ariel Lecca and Mike Bettlestone, who had previously announced their plan to retire from their respective Committee leadership positions at the end of our meetings. Together, Ariel, the leader of the ISO Standards Committee, and Mike, the leader of the CISPR D Standards Committee, contributed nearly 50 years of active participation to the work of these committees, and a special celebration was in order.
During a convivial celebratory meal on the last day of our meetings, we shared humorous stories about Ariel’s and Mike’s involvement over the years, took several photos, exchanged warm handshakes and hugs, and shed a few tears in the process.
The celebration was our small way to recognize and honor the dedicated leadership of Ariel and Mike. Their intelligence and tenacity, combined with their diplomacy and sense of humor, helped guide our work on these important automotive EMC standards, allowing us to continuously adapt these standards to the latest technologies while also maintaining the utmost integrity in EMC measurements.
And so, to Ariel and Mike, thank you for expertly guiding the ISO and CISPR D Automotive EMC Standards Committees for many years! Your legacy will not be forgotten!