A New Method for Oscilloscope-Based Power Integrity Testing
With the continual decrease in power rail DC voltages and tolerances, oscilloscopes remain a key tool for development teams tasked with power integrity measurements. The continual trend to lower voltages and tolerances has driven the proliferation of power rail probe usage with oscilloscopes. The question of “should we purchase our first power rail probe” has changed to “what’s the best measurement technique”. Initial techniques have been refined as users and oscilloscope manufacturers collaborate and share insights and experiences.
A relatively unknown yet superior technique emerged from experts making power integrity measurements. This approach involving histograms and free-run trigger mode offers three key improvements compared to the traditional approach:
- By using a waveform histogram, users can visually and statistically compile power rail attributes;
- By using a single histogram measurement across all acquired waveforms instead of a measurement on each waveform, speed-of-test is accelerated, and with great accuracy; and
- By using free trigger mode, users get increased real-time power rail coverage versus instrument dead time when the instrument is blind to power rail signal activity.
All major oscilloscope manufacturers offer power rail probes, and these probes incorporate several attributes that make them superior for highly accurate measurement of small voltages with tight tolerances. Lots of material is available on the benefits of using power rails. This includes the probes’ minimal DC loading impact on the power rail, built-in offset to enable users to take advantage of small vertical scaling, and a 1:1 attenuation ratio to minimize noise. The relatively new histogram approach to power rail measurements accelerates test time by more than 50 times while providing more accurate measurement results.
Traditional Approach
The long-used approach for measuring power rail tolerance is to have the oscilloscope use a voltage peak-to-peak measurement to determine the overall amplitude from noise, ripple, and periodic disturbances (see Figure 1).
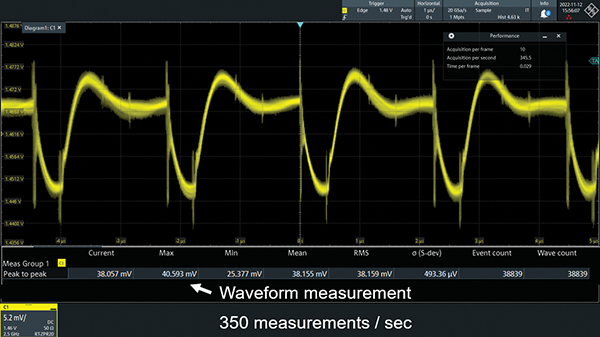
Oscilloscope users agree that a key issue with this traditional approach continues to be oscilloscope blind time. All oscilloscopes are subject to blind time between acquisitions. During this time, the oscilloscope is processing the last acquisition and cannot perform any new acquisitions until the previous one has been processed. While engineers are generally aware of this, many are not aware that the ratio between blind time and real-time signal acquisition can be extremely large. It is not uncommon to have 1000 times the amount of blind time compared to acquisition time.
Several attributes related to testing power rails lead to additional undesired blind time. Oscilloscope users never achieve the faster update rate needed for better testing and hence are only able to test snippets of time, while missing large amounts of rail signal activity between acquisitions.
Oscilloscope manufacturers communicate a waveform update rate (wfms/s) for specific oscilloscope families. This value describes the maximum speed at which the instrument can acquire and display signals. The fastest oscilloscopes in the world have a waveform update rate in excess of one million waveforms per second, while slower ones will have an update rate in the tens of waveforms per second. Waveform update rate describes a maximum value, but this value is not typical for power integrity measurements.
Maximum update rates occur at a specific time base setting, with the fastest sample rate, and without any measurements turned on. However, this is not descriptive of a power integrity test setup. At time bases of 1 uS/ and slower that are typically used for power rail measurements, blind time increases.
Testing power rails varies from other types of testing in that a trigger signal is not always readily available. Most oscilloscopes require a minimum voltage swing for detecting a trigger event. Power rails often do not have enough signal swing and hence users choose auto-trigger, which means if the oscilloscope does not find a suitable trigger it will table an acquisition after a predefined period of waiting, typically a few mS. This triggering issue also contributes to users missing significant time when the oscilloscope is not acquiring power rail activity. Alternatively, in normal mode when the oscilloscope is triggering on the signal, it still needs to re-arm the trigger after each acquisition, which in turn slows down the waveform update rate.
Lastly, turning on measurements on all oscilloscopes reduces the instrument’s acquisition rate. An oscilloscope that acquires 1 M waveforms per second without any measurements on might have a reduction to 350 acquisitions per second when measurements are turned on. An oscilloscope that normally acquires 50 waveforms per second might be slowed down to 5 acquisitions per second when a measurement is enabled. The need to process a measurement results in additional time during which the instrument is not actively acquiring power rail activity.
How much power rail activity do oscilloscopes miss? It is not uncommon for an oscilloscope with a fast update rate, a time base setting of 1 uS/div, and a single waveform measurement to have an update rate of 300 to 400 measurements per second. Is this fast? This update rate and time base combination means the oscilloscope is missing 99.6% of real-time power rail activity. Any anomalies that occur on the power rail during this blind time will not be recognized or measured by the oscilloscope. This processing speed tops other oscilloscopes where the update rate is just a few measurements per second, resulting in missing > 99% of power rail signal behavior.
Peak-to-peak voltage measurements are made on each individual waveform with results accumulated across multiple acquisitions. This provides a peak-to-peak range for each individual waveform, but it does not incorporate the largest maximum value versus the smallest minimum value that is acquired over successive acquisitions. What is needed is a peak-to-peak measurement that encompasses all acquisitions and updates as the oscilloscope acquires new power rail signal activity.
A Superior Approach
Several modifications to the traditional approach enable users to dramatically increase test time coverage and achieve more accurate measurements. The improved measurement techniques build on less frequently used oscilloscope settings that have existed for a long time, but that have not been exploited collectively for a specific application. Power integrity happens to be the key application where these capabilities combine to produce a result far better than the traditional power integrity measurement approach used by most engineers today.
1. Use Waveform Histogram
A number of currently available oscilloscopes offer a less commonly known feature called waveform histogram (see Figure 2). The instrument computes all waveform values and produces a corresponding histogram that shows what vertical values the waveform had, and what percentage of acquisition samples were at a specific amplitude. In a sense, histograms are very compact representations of waveform amplitude values at each sampled point. The histogram does not retain the detail related to the shape of each waveform, but rather just the vertical values. This is exactly what is needed for power integrity measurements.
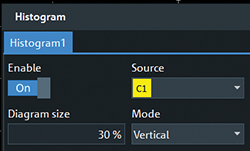
Figure 3 shows a waveform histogram of a power rail. From the histogram, the user can quickly determine how much of the time the signal under test spends on each level. The histogram incorporates information from all acquired samples in each acquisition and builds up with each successive acquisition.
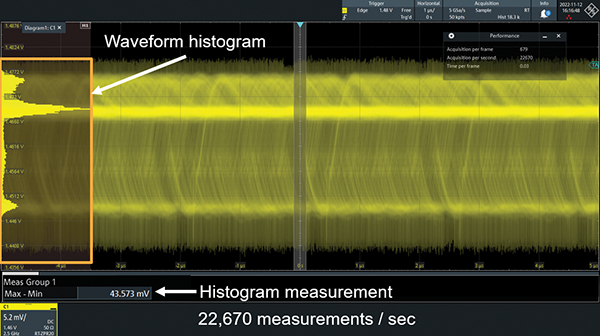
Why use a waveform histogram? For voltage tolerance testing, the shape of the waveform is not important. What is important are the minimum and maximum values. Rather, ripple, noise, and disturbances present themselves as anomalies that exceed voltage tolerance levels. A histogram is a great visualization tool to see if tolerance levels have been violated.
For many oscilloscopes, waveform histogram processing is done in hardware, and the oscilloscope experiences little to no drop in the maximum waveform update rate. With waveform histograms, such instruments can measure 20 times more acquisitions per second versus using a Vpp measurement on each acquisition. Such oscilloscopes capture as much as 20 times more real-time signal activity on the rail.
2. Make the Peak-to-Peak Voltage Measurement (Vpp) on the Histogram
Apply Vpp, min, or max measurement to the waveform histogram instead of measuring each individual waveform. Why is applying a Vpp measurement to a waveform histogram better than applying to individual waveforms? Since the waveform histogram contains power rail waveform information from all past acquisitions, the measurement applies to all acquisitions. For example, if a histogram is composed of 1000 repetitive acquisitions, a single peak-to-peak measurement on the histogram covers the composite information from all 1000 acquisitions. But the oscilloscope only needs to make a single measurement, instead of 1000 measurements, meaning the oscilloscope’s update rate stays faster.
In addition to the speed increase, the peak-to-peak measurement on the histogram is made across all acquired data. It constitutes the true max peak-to-peak voltage from the highest value versus the smallest value. This provides the correct overall peak-to-peak value overall acquired data, versus the less accurate traditional approach that measures just the worst-case single acquisition that may not include the highest maximum voltage and the lowest minimum voltage of all acquisitions.
3. Use Free Run Trigger Instead of Auto or Norm Trigger
The third technique to achieve a more comprehensive, hence more accurate, power integrity test on power rails includes a change in how engineers trigger their oscilloscope. For testing rail voltage tolerances, triggering on a specific part of the waveform is not required. In fact, in many cases, oscilloscopes require larger voltage swings than occur on power rails. Because of this, testing of power rails is often done with the oscilloscope set to the default auto-trigger mode. If the oscilloscope does not find a trigger within a short period of time, typically a few mS, it goes ahead and acquires. The auto trigger mode inherently slows down the oscilloscope acquisition rate, meaning testing includes a small portion of the power rail real-time signal activity. Switching the trigger mode to norm yields a similar update rate as the auto trigger.
For power integrity tolerance testing, a trigger on a specific part of the waveform is not critical. Many oscilloscopes incorporate a less common trigger mode known as free run (see Figure 4). With the trigger set to free run, the oscilloscope captures data, processes the data, and then captures the next acquisition without having to look for or wait for a trigger event. The tradeoff is that the trigger does not occur at the same point of the waveform each time. For power integrity tolerance testing, triggering on the same part of the signal each time is not needed and there are benefits in asynchronous triggering. Free run mode yields a waveform update rate dramatically faster than in auto or normal trigger modes. For example, on a specific oscilloscope tested with a time base setting of 1 uS/, in auto trigger mode the capture rate is 1,200 wfms/s while in free run the acquisition rate increases to 22.7 K wfms, almost 20 times better.
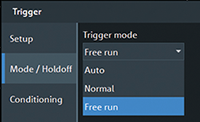
Table 1 shows the difference between the traditional measurement-per-waveform approach, versus the refined histogram combined with free-run trigger mode. (An R&S model RTO6 oscilloscope was used to highlight the differences between the two approaches.)
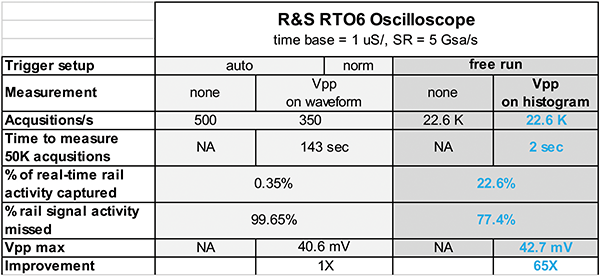
Like many approaches, combining multiple techniques yields significant advantages. For power rail testing with oscilloscopes, using waveform histograms, plus measurement on histograms, plus free run trigger mode enables users to capture and analyze a significantly higher percentage of rail activity than the traditional method of making peak-to-peak voltage measurements on individual waveforms with the oscilloscope’s trigger set to auto or normal mode.