Developments in Ground Bonding Networks
Editor’s Note: In this article, the words “ground,” “grounded” or “grounding” are used interchangeably with “earth,” “earthed,” or “earthing.”
EMC1 for Systems and Installations
As the quantity and variety of electronic equipment used in systems and installations continues to grow, EMC is becoming an increasingly important issue. Almost all my work on systems and installations since 1990 has been involved with items of equipment interfering with the correct operation of other items on the same site.
In 1990, variable speed motor drives using insulated gate bipolar transistors (IGBTs) and power field-effect transistors (PowerFETs) for high-speed power switching were very new, as were private mobile radio systems, and they both caused many problems with legacy electronic equipment. Since then, EMC standards and regulations in most countries have considerably improved the emissions and immunity of equipment, but at the same time, variable speed motor drives have constantly improved – by switching faster. Faster switching makes them more efficient, smaller and less costly, with the result that they are being used much more widely. Unfortunately, switching faster causes increased noise emissions at higher frequencies, increasing the possibilities for interfering with other equipment (see Figure 1).
The systems and installations concerned are not just land-based, because all new marine and submarine vessels now use electric motor drive technologies exclusively. They still have huge marine diesel engines, but they drive huge electricity generators rather than being directly connected to propellers. Electric automobiles are already proven in use and a growing industry, of course, and all-electric aircraft are on the drawing board.
Another big technological leap in switching power converters is happening right now: replacing IGBTs and PowerFETs with high electron mobility transitors (HEMTs), usually based on Gallium Nitride, GaN, and with silicon carbide (SiC) PowerFETs, which can switch efficiently at ten times (or more) higher rates, reducing size and cost even more.
This is all good news for improving power efficiency, saving on energy bills while also helping to save the planet by reducing emissions of CO2. But it comes with the big EMC penalty of higher levels of noise emissions at higher frequencies, as Figure 1 illustrates.
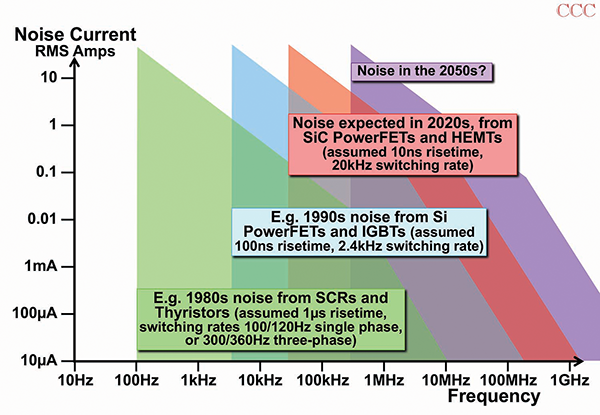
Many higher-power switching converters such as variable-speed motor drives are supplied with installation instructions concerning EMC, which are usually quite good. However, my experience (and the experience of others in the EMC world) is that salespeople compete on price by ignoring the EMC installation requirements of new motor drive systems to help win contracts with customers who do not realize the EMI risks.
No one wants to invest in the construction of a new industrial plant, scientific research facility, offshore oil/gas platform, railway system, entertainment complex, data center, military vessel, cruise ship, or whatever, only to find that it cannot function properly due to self-generated EMI! The financial losses can be quite awesome, but even they can be exceeded by the cost of modifying the plant, platform, vessel, etc., to get it to function as intended.
As with all other EMC/EMI issues, it is much more cost-effective and financially less risky to design good EMC in from the beginning of any modern construction project.
Tim Williams and I co-wrote a book on EMC for Systems and Installations in 2000 [1]. It describes buildings and sites, but its material is easily extended to cover vehicles of all/any types, land, marine, subsea, air (fixed wing or rotorcraft), space, etc.
Among many other issues, this book covers the design and construction of the various kinds of (so-called) “earthing/grounding” systems/networks used in large systems and installations, including:
- Bonding networks (BNs)
- Isolated bonding networks (IBNs)
- Common bonding networks (CBNs)
- Meshed bonding networks (MESH-BNs)
- Meshed isolated bonding networks (MESH-IBNs)
- Meshed common bonding networks (MESH-CBNs)
This two-part article provides some background on these “grounding networks” that is not given in the book. It shows how and why, over the last few decades, they have had to develop from their original single-point-grounding schemes for safety, to meshed structures for cost-effectively managing EMC to minimize the costs of lost production and downtime due to EMI, including lightning.
The Protective Equipotential Bonding System
Metal and other conductive structures in buildings and vehicles, including all wiring and cables, can suffer from fault currents caused by insulation failures in their power supply equipment and distribution networks, and from surge transient currents caused by lightning.
As fault or surge transient currents flow through the impedances of these metal structures and other conductors, they create heat and potential differences that could cause burns and electric shock hazards
to people.
In flammable or explosive atmospheres, high temperatures could cause fires or explosions, as can the arcs or sparks that can be created when potential differences appear across gaps.
In IEC safety standards terminology, a protective equipotential bonding system is the total conductive structure created by interconnecting all of the “touchable” metal parts that could cause electric shocks to people due to insulation failures or the indirect effects of lightning2 (see Figure 2).
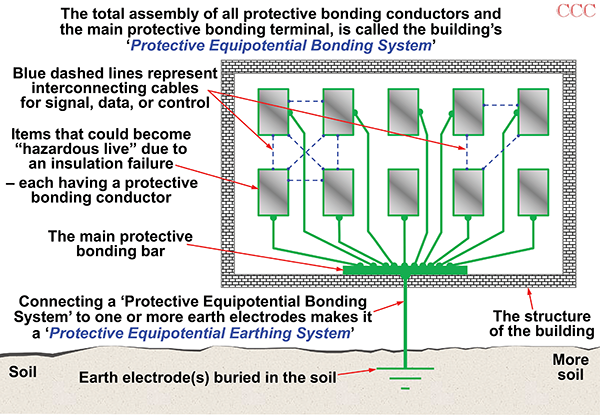
“Touchable” means metal/conductive parts that are within the simultaneous reach of any parts of a person’s body. This includes, for example, equipment cabinets or other conductive structures on either side of a walkway that are close enough to be touched by the fingertips of a person’s left and right hands at the same time.
“Equipotential” is usually considered to mean – for dry environments – that the maximum continuous potential difference is no more than 25VAC rms at the 50Hz or 60Hz mains power frequency, or no more than 60VDC. Higher maximum values may be permitted for short-term potential differences and/or lightning-induced surge transients.
It is important to understand that the above values are general, and the actual values considered safe enough can vary from one standard to another, and from one country to another, and are usually specified at much lower values where environments can be wet or humid or skin could be sweaty, because human skin resistance is lower in such situations.3
It is often forgotten that “touchable” also includes the shells of mating connectors, and shielded cables that extend for many metres effectively extend a person’s reach by the length of the cable! A good friend of mine fell from a very tall ladder when he disconnected an audio cable from a ceiling-mounted amplifier in a theatre and – because one hand was on the amplifier and the other on the disconnected cable’s metal connector – got a severe electric shock. The fall broke his leg, and at that he was very lucky.
The maximum potential differences permitted for “equipotentiality” are achieved by ensuring sufficiently low impedances throughout a protective equipotential bonding system. The series impedance (Z) of a long conductor (well below its first quarter-wavelength resonance) is:
Z = √[R2 + (2πf L)2]
Where:
R = series resistance in Ohms
L = series inductance in Henries
f = frequency in Hertz
For 50Hz and 60Hz, the impedance of most practical conductive structures is dominated by the R term in the above equation. However, most of the energy in lightning surge transients lies in the region of the spectrum up to 1MHz, and above a few kHz most conductor’s Zs are dominated by the 2πf L term. So, the series impedances of ordinary conductors (e.g. those used for bonding items of equipment in protective equipotential bonding systems) become predominantly inductive above a few kHz, which is important for lightning protection (and for EMC, see later).
Mains power supplies are always protected by overcurrent protection devices, such as fuses. In the event of an insulation failure to the protective equipotential bonding system the resulting fault currents are so much higher than the normal mains currents that the overcurrent protection operates to remove the power from the conductor with the faulty insulation, generally within 1 second.
Metal structures and conductors in correctly designed, constructed and maintained protective equipotential bonding systems have low-enough resistances not to cause electric shocks. This almost always means that all their constituent parts have sufficient conductor mass and cross-sectional-area (CSA) to ensure that, in the time it takes the overcurrent protection to operate and stop current flowing from the faulty part or conductor, none of their parts will become heated up by enough to burn people’s skin. Usually, the worst-case rise in temperature might just feel to the hand like a slight increase above ambient temperature.4
Indirect lightning surge transient currents could be as high as 10s of kA, but their durations are so short, at a few microseconds, that their heating effect in properly constructed protective equipotential bonding systems is also negligible. However, this is not necessarily the case for Lightning Protection Systems (LPSs).
Where flammable or potentially explosive atmospheres may be present some or all of the time, there are additional requirements for “equipotential bonding” to reduce the maximum potential differences between different metal parts. This is not to reduce electric shock risks, but to reduce the risk of sparking causing fire and explosion hazards. These are not covered here, but they are very well specified in the relevant standards.
For buildings on land, it is usual to connect this interconnected bonding structure to ground electrodes (conductors buried in the soil under and/or around the building) thereby creating a “grounded protective equipotential bonding system.” 5
It is important to understand that grounding (i.e., connecting to ground electrodes) is not always necessary, or desirable to protect against electric shock hazards. For example, even vehicles with on-board 230V AC mains distributions don’t need grounding (in the strict sense of the word) to be safe. And trying to provide them with direct connections to ground electrodes would not be very successful!
In fact, the only reason for connecting a protective equipotential bonding system to the soil under and/or around a building is so that if people are stepping into or out of a building at the same moment as a nearby lightning strike to ground, or to the building, the surge transient voltage that is created between the building and its surroundings (e.g., sidewalks, roads, steps, stairs, gantries, etc.) is not so high as to cause them injury due to electric shock.6
In all non-medical equipment that complies with IEC safety standards for 230VAC rms mains supplies, the mains leads and the mains power converters are both insulated and galvanically isolated to withstand overvoltages caused by lightning surge transients up to at least 3kV rms, 4.24kV peak, repeated for many years. (Medical equipment safety standards generally require higher withstand voltages.)
A long, long time ago, electrical and electronic equipment was just a box on the end of a mains lead. For such equipment, the protective equipotential bonding system, grounded or not according to whether it was fixed or mobile, was sufficient.
However, signal/data cables connecting different items of equipment within an installation can be long, which exposes them to much larger potential differences. When such cables started to be used more often to interconnect items of equipment that were further apart, the cost, size and weight of insulating and isolating them to the same levels as used for mains cables was considered to be too high. So, bonding networks (BNs) were developed.
Unreliability of electronics due to the effects of lightning (within 5km or so!) is, for example, the reason why Ethernet transmitters and receivers always use data-isolating transformers – so that people could easily install Ethernets in legacy buildings which had protective equipotential bonding structures like those shown in Figure 2 (long wires from each piece of metal all the way back to a main bonding or grounding bar). These types of legacy installations suffered from high impedances above a few kHz, making them unable to control the lightning surge transient voltages that could occur between two items of equipment connected by a signal/data cable.7
Bonding Networks (BNs)
This is IEC-speak for a part of a protective equipotential bonding system that uses additional cross-bonding of its conductors and other metalwork to reduce its overall impedance, so that the amplitudes of the high voltage surge transients that can arise between items of equipment that are located far apart, caused by lightning, are low enough to permit the use of affordable insulation/isolation on signal and data cables (typically, half of the peak voltage required for the insulation of mains supplies, and without the mains supply’s requirement for galvanic isolation.)
To achieve a low enough inductive impedance up to 1MHz requires the lengths of the bonding conductors to be less than a metre. This can’t be achieved by relying on the protective conductors in the equipment’s mains leads, because the main bonding (or grounding) bars these conductors eventually connect to are often ten or more metres away, even in a small building like a domestic house. In an office block or industrial plant, the distances can be several tens of metres.
We sometimes see the impedance of conductors at the frequencies associated with lightning events referred to as the “surge impedance.” This is a time-domain concept based on the surge test waveforms created by “combination wave generators” such as those specified by IEC 61000-4-5. Surge impedances are not relevant for EMC at frequencies above a few MHz.
Cross-bonding conductors and other metalwork has a strong effect on reducing the inductive impedances in a BN. To get the best protection for the lowest costs, the cross-bonding conductors should follow the routes (ideally, be strapped to) the signal/data conductors themselves, as shown in Figure 3.
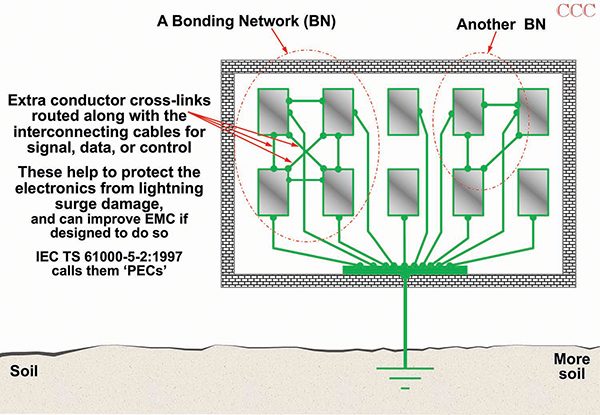
IEC 61000-5-2 [3] calls cross-bonding conductors that follow the routes of the signal/data cables “protective earthing conductors” (PECs), and describes how existing structural metalwork, often called “natural metalwork,” may be used to reduce the costs of implementing them. For example, in industrial and commercial applications, cables are usually supported by metal cable trays and conduits, which can be converted to very effective PECs merely by electrically bonding them together and to the chassis/frames of the different items of equipment whose cables they carry.8
Usually, a BN comprises a single room in a building where there are signal/data cables that need protecting because of the electronic equipment contained in that room. Where there are two or more individual BNs they are interconnected, at least by individual connections to the main bonding bar, as shown in Figure 3. (If the main bonding bar is used to connect the BNs directly to ground electrodes buried in the soil under and/or around the building, it is called a “main grounding bar.”)
A question that always arises is whether the cross-bonding conductors have to be rated to carry the full fault currents that can arise due to insulation failures in the 50 or 60Hz mains power supplies and distribution networks. The answer is that, in cases in which a protective equipotential bonding system complies with modern safety requirements (and is maintained so as to ensure continual compliance) so that during such faults, it does not overheat sufficiently to suffer damage including to any insulation, then cross-bonding will not have to be rated to carry mains fault currents. Even cable shields electrically bonded at both ends (the only way to make them shield properly at RF, see [1], [3]) should not be damaged by a mains fault in a correctly-bonded protective safety system.
However, many legacy protective equipotential bonding systems might not meet modern safety requirements without extensive and costly modifications, and some owners or users of older buildings are known to use a “there is no evidence of a problem” approach to avoid such costs.
When asked to work for such an owner or user, I strongly recommend turning down the work unless you are allowed (and paid!) to do a “proper job” safety-wise. Never underestimate the ability of legal counsel to pin the blame for a costly or lethal fire or electrocution on anyone other than their client, even going against common sense that “everyone knows.”9
Over time, systems have grown larger, variable speed drives have become larger and more common, and systems-of systems are now being created, meaning that signal/data cables have to span two or more BNs (e.g., computer networks, lighting control systems, air-conditioning systems, etc.). These BNs therefore have to be cross-bonded together to create larger BNs, ultimately cross-bonding the whole building and creating a common bonding network, or CBN.
But CBNs can be costly to retrofit to legacy buildings or vehicles, so the BN concept was developed to create the IBN – isolated bonding network. We’ll discuss that in Part 2 of this article.
Endnotes
- EMC = Electromagnetic Compatibility, the engineering discipline of ensuring that: a) electromagnetic emissions are low enough for radio/telecommunications and other electronic equipment to function as intended without suffering from unacceptable electromagnetic interference (EMI); and that, b) the electromagnetic immunity of equipment is sufficient for it to function as intended in the electromagnetic environment expected to be present where it is used. Note: EMC standards and legislation are not concerned with any safety issues, despite the fact that EMI can be a cause of functional safety risks. For a number of articles on this topic, visit https://www.emcstandards.co.uk/emiemc-risk-management.
- Protection from the direct effects of lightning to buildings themselves and the people and equipment within them, is provided by a Lightning Protection System (LPS), e.g. as specified in the four parts of IEC 62305. An LPS is a different structure from a Protective Equipotential Bonding System, although the two structures are usually connected together at one or more points. LPSs generally are designed to withstand surge transient currents of the order of 100kA – 200kA and are not covered any further in this article, but see Chapter 9 in [1] for information on Part 4 of IEC 62305, which deals with protecting electronic equipment rather than people and is relevant for the design of Protective Equipotential Bonding Systems.
- 25VAC rms and 60VDC are both far higher than what would be understood as “equipotential” by electronic engineers, showing one of the dangers of misusing safety terminology in other applications. To help avoid costly confusion in projects, I always recommend only using the word “equipotential” for issues relating to personnel safety from electric shock, and never for anything to do with electrical or electronic circuits, or EMC.
- However, there are some power supply systems for which this might not be true, and for which the temperature rise in the protective equipotential bonding system might need to be controlled to prevent burns, such as in installations that use large superconducting magnets. Large grid-storage batteries are another modern concern.
- Regular readers of my blogs [2], or people who have attended my training courses are used to me banging on about the very costly confusion caused by the misuse of the words ground, grounded, grounding, etc. Strictly speaking, they should only ever be used for conductors that connect directly to the body of the planet by being buried in the soil. But people use them indiscriminately, e.g., they talk about “grounding” an electrical circuit in a cellphone; or about “safety grounding systems” in cars and aircraft, when none of these examples has anything to do with the soil, or any need to be grounded (in the strict sense) for either correct circuit operation or safety. I’m not the only one who complains about the misuse of terminology associated with “ground.” Check out “The Ground Myth” by Dr. Bruce Archambeault of IBM, especially slide 37 for a good laugh.
- This reminds us to be careful when stepping into or out of vehicles during thunderstorms! The best method is to jump in, or out, with both feet at once, keeping both hands and arms tight in against our sides. However, I should mention that an alternative method – simply trusting to luck that lightning won’t strike the vehicle or near to it while getting in or out – is preferred by most people. Can’t think why!
- Telephone landlines are a special case because they do pick up lethal kV surge transients from lightning due to their very long lengths exposed outside of buildings. Telephone systems providers learned very early on to provide suitable protection, at the point where their landline entered/exited a building, because killing off your customers and/or setting fire to their houses is not good business sense.
- I really wish they hadn’t called them protective earthing conductors, because their protective function works just as well whether they eventually connect to earth electrodes or not. They should really have been called “protective bonding conductors” (PBCs), or “supplementary bonding conductors” (SBCs). IEC 61000-5-2 [3] is a truly excellent document in every way – a total game-changer in the 1990s (that most system integrators and installers still don’t seem to know about) – except that it uses the words “earthed” and “earthing” when it really means “bonded” and “bonding.” See my little rant earlier about the costly confusions still being caused by this age-old mistake.
- It seems that even Ohm’s Law can be a matter for juries (who are almost always non-technical people) to decide upon!
References
- EMC for Systems and Installations, Tim Williams and Keith Armstrong, Newnes 2000, 0-7506-4167-3.
The practical material in the book is also available in a series of articles in 2000, two free guidebooks posted here and here, and the up-to-date training course. - Keith’s blog
- IEC 61000-5-2:1997, “Electromagnetic Compatibility (EMC) – Part 5: Installation and mitigation guidelines – Section 2: Earthing and cabling,” from the BSI and IEC web shops.