Understanding Radiated Emissions Measurements Made at One-Meter Separation: It’s Not What You’ve Been Led to Believe
This is the final installment of this serialized article. The first installment explained what near and far field measurements entail, and that one-meter measurements are very much near field. The second installment explained the evolution of the earlier 12” and present-day one-meter separation measurements, considerations in antenna selection, the difference between antenna-induced and field strength limits, and the evolution from one to the other. The third installment investigated practical problems arising from the misapplication of field intensity and far-field concepts to near-field phenomena.
This fourth and final installment lists theoretical misunderstandings arising from the erroneous substitution of field intensity for antenna-induced concepts and shows the serious practical outcomes that result from these theoretical mistakes.
Theoretical Problems Resulting from Using Field Intensity Limits Where Antenna-Induced Is More Appropriate
Ignorance of the fundamentals of extreme near field measurements and of gain derating and the nature of the Fresnel zone has pronounced and dangerous impacts on both EMI and EMC requirements and verification. The following sections provide some relevant case histories.
Conflating Radiated Emissions and Radiated Susceptibility
With radiated emission and radiated susceptibility requirements both having field intensity limits, the idea that radiated emission (RE) and radiated susceptibility (RS) control were somehow two sides of the same coin became prevalent. People claiming that one controls RE to avoid RS was enough of a problem that in 1993 instructions were included in MIL-STD‑461D specifically saying that this belief is not true and that, in fact, RE limits protect antenna-connected receivers and RS limits provide a level of immunity to intentionally generated RF fields from high-power RF transmitters. These instructions have remained in all subsequent revisions of the standard.
Although the RE/RS comparison is extremely damaging, it is still assumed to be true by many engineers, often in the space community.29 It is easy to see why: the space community doesn’t typically use the rf spectrum below several hundred megahertz, so there is no need for RE limits at lower frequencies, and often no or rudimentary RS limits there. Since they have used military limits covering as low as 10 kHz without comprehending the real need for such, they substitute this incorrect duality to justify using incorrect requirements. This leads to two errors. One is complete disrespect for the discipline, since comparing stringent RE limits to even the most rudimentary RS limits shows “margins” on the order of 100 dB.
The second error is to attempt to show the “margins” aren’t that high because a culprit (unintentional) emitter source may be placed very close to an (unintentional) victim receiver, requiring “scaling” of the one-meter RE limit to a distance of just a few centimeters. Such “scaling“ is done using Hertzian dipole field intensity behavior vs. distance, typically third order (cube of distance ratio).30 Such comparisons are not accurate in the extreme near field, since the Hertzian dipole derivation assumes the observation distance is large with respect to the radiating structure dimensions – clearly not the case a meter away from a two-meter-long test sample.
While Hertzian dipole equations are often applied to various EMC-related problems and analyses, it is not difficult to see that they are inapplicable or at best only partially applicable to one-meter radiated emission measurements. One need not wade through the several-page derivation in Reference 30. The inapplicability is at the very beginning, where the expression for the magnetic vector potential is derived.31 The requirement that the distance to the observation point be large with respect to the dimensions of the radiating element is inherent in the Hertzian expression for the magnetic potential vector, from which the entire derivation proceeds. It should not be surprising that the Hertzian dipole field expressions all “blow up” close to the source. The simplifying assumption that the distance to the observation point is large with respect to the dimensions of the radiating element means that the radiating source is a point. If there is current (flow of charge) on a point source, charge separation and potential differences follow.
Reference 24 (presented in the September issue of In Compliance Magazine and referenced in Part 3 of this serialization) derives and shows that, in the direction of maximum radiation, the Hertzian expression for the electric field is off by 3 dB when the separation distance is twice the dipole length, and that the electric field in closer than that approaches a fixed level proportional to the charge separation divided by the dipole length. When the observation point separation is one-tenth the dipole length, the Hertzian expression is 60 dB too high.
Since 1993, MIL-STD-461 has included in the CE102 limit rationale the information that, in the rod antenna range, the relationship of the measured radiated field to the voltage on a 2.5-meter-long wire is such that the wire potential is 40 dB higher numerically than the radiated signal measured. Reference 32 provides analytical details.32 If the wire potential is 40 dB above the signal measured a meter away, and the wire is suspended 5 cm above the ground plane (-26 dB above one meter), that makes the field intensity between the wire and ground plane 66 dB higher than at one meter. That is an upper bound on such scaling.
A shorthand model for this is shown in Figure 4 in Reference 24. One end of the dipole is now a wire suspended 5 cm above the tabletop ground plane and its image below the ground plane is the other end, for a net dipole separation of 10 cm. Looking at the circled point on Figure 4 of Reference 24, the field falls off beyond 20 cm from the wire as the cube of the distance ratio. So, from 20 cm to 1 meter away, the field falls off by a ratio of 53, or 42 dB. From 20 cm away from the wire to the wire, the field intensity increases by about 20 dB. The ratio of the field intensity between the wire and the ground plane beneath it to the field intensity found at a point a meter away is 62 dB. That calculation is for a pair of separated charges. The answer is within a few dB of the exact calculation for a cable of significant extent, measured not at a point but using a 41” rod antenna. Not bad, and it serves to dispel the mistaken superstition that Hertz says things should blow up.
It should be noted that this is not about radiated energy at all. This is the fringing field from a source of charge separation. In this model, the only current that could contribute to actual radiation is the displacement current between the wire and the ground plane, and using a value such as 10 pF/m yields next to no current over most of the rod antenna frequency range. The fringing field dominates, and it dominates even more so at 12” than at a one-meter separation. Citing NADC-EL-5515:
“In general, the ratio of the electric to the magnetic components surrounding an unshielded lead will vary directly as the impedance of the load terminating the lead, and the apparent impedance presented to the various pick-up antennas will vary in the same manner. This statement applies to radial and tangential field components as contrasted with the more usual concept of wave impedance encountered in shielding theory, which applies only to the components tangential to the line of propagation.”
One-Meter Measurements Are Not Scalable Outwards, Either
Similar to the conflation misconception just described, people attempt scaling the one-meter results to a larger distance. Figure 13 portrays this type of an error. Some people advocate changing the one-meter measurements to three meters or more, based on the assumed accuracy superiority of far-field measurements. Some advocate for including the option of three-meter measurements in lieu of a one-meter measurement. The erroneous assumption is that one can adequately replace the other. As explained earlier, there are two errors here: 1) the radiation source is not a point source at either one- or three-meter distance; and 2) the one-meter measurement is not the field at some point in space but the average over the physical dimensions of the EMI test antenna. So not a scalable value in any way, shape, or form.
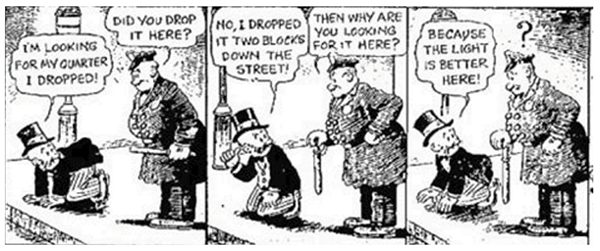
Misapplying Antenna Factors
SAE ARP-958E (2021) now provides for separate horizontal and vertical polarization antenna factors for dipole-like antennas (dipoles, biconicals, log-periodic arrays). Under the right conditions this might make sense (given the a priori use of field intensity limits in the extreme near field) but it is indefensible given how they measure antenna factors.
The 1968 release SAE ARP-958 was for the newly mandated log spirals of MIL-STD-461.33 SAE ARP‑958 did not specify the height of the antennas above the floor, nor even if the floor would be reflective or not. But SAE ARP-958A (1992) specified a reflective floor and a three-meter height above the floor.34 The purpose was to control ground plane effects and make them negligible relative to the direct coupling between transmit and receive antennas. All subsequent revisions maintained the three-meter height and the reflective ground plane.
Note that, while all one-meter separation radiated emission requirements (CISPR 25, RTCA/DO‑160, and all revisions of MIL-STD-461/-462) place the antennas approximately one meter above the ground plane, SAE ARP-958E measures the separate polarization-based antenna factors three meters above the ground plane. Ground plane effects measured at three meter height are not even remotely applicable when antennas are one meter above the ground plane. Consider that over fifty years ago, just a few years after the biconical antenna was introduced in MIL-STD-461 (1967), a handbook warned that placing the lower tip of a vertically oriented biconical within two feet (61 cm) of the floor would capacitively load it sufficiently to change the antenna factor.35
The point is this: if you want the biconical placed with the lower tip one foot above the floor (CISPR 25), then fine, make it so. And make everyone do it the same way. But then don’t apply a correction factor measured at three meters above the ground to correct for that. Don’t correct for it at all. Just mandate the proper antenna, and its separation and orientation, and then record antenna output, adjusted for balun and cable losses.
Misapplying Far-Field Gain in Computing Power Density
The EMC departments of two aerospace vehicle manufacturers refused to perform system-level EMC verifications that required the use of RF absorber panel emplacements around their respective platforms. They based their decisions on the belief that the computed RF power density from a platform high-gain microwave dish exceeded the absorber power rating. This was based on computing the power density using the far-field gain (only achieved beyond 50 meters distance) at a distance of two meters from the dish.
In reality, the actual power density based on Figure 12 (in Part 3) was orders of magnitude less and much less than the absorber panel rating. Further, an elementary calculation of the area over which the far-field gain would concentrate the beam at a two-meter distance would have been a few centimeters on a side, which should have been a tip-off. Another tip-off would have been a Friis equation calculation showing more received power than transmitted using the far-field gain close in.
Basing RE Limits on the Far-Field Gain of a High‑Gain Dish
A non-US space agency levied a millimeter wave radiated emission limit based on the far-field gain of a high-gain dish. The resulting limit was such that it could not possibly be instrumented. The Fresnel-zone gain of such a dish at a one-meter distance is again orders of magnitude less than in the far field, plus the potential noise sources are in the back lobes of the antenna or, in a worst-case scenario, a 90-degree side lobe, but certainly not in the main beam.
Antennas Are Not Interchangeable in the Near Field
Both RTCA/DO-160, section 21, and CISPR 25 recommend but do not require the use of standardized antennas for radiated emission measurements. This is a direct consequence of using field intensity limits, and the assumption that the use of antenna factors is enough to yield the proper result no matter what antenna is chosen. The 1995 release of CISPR 25 went so far as to claim:
6.5.1 Antenna systems
“The limits … are listed in dB(uV/m), and thus theoretically any antenna can be used, provided that … the antenna correction factor is applied…”
This type of error was identified in 1967 when MIL-STD-461 popularized the change from antenna-induced to field intensity limits.36 The 2016 edition removes the egregious statement but only recommends standard antennas. In this regard, all versions of MIL-STD-461/-462 RE02/RE102 since 1967 are superior to RTCA/DO-160, section 21, and CISPR 25 in recognizing and accommodating the near-field nature of the measurement at a one-meter distance. The 41” rod antenna and 137 cm tip-to-tip length biconical have been required since 1967. From 1967 – 1993, log spirals were required from 200 MHz to 10 GHz. After 1993, the log spirals were replaced by double-ridge guide horns of specified aperture dimensions. Since 1993 the following rationale appendix wording explains the use of standardized antennas for requirement RE102:
“Specific antennas are required by this test procedure for standardization reasons. The intent is to obtain consistent results between different test facilities.
“In order for adequate signal levels to be available to drive the measurement receivers, physically large antennas are necessary. Due to shielded room measurements, the antennas are required to be relatively close to the EUT, and the radiated field is not uniform across the antenna aperture. For electric field measurements below several hundred megahertz, the antennas do not measure the true electric field.”
The first clause of the very last sentence is unnecessary since even if the calculated far field of the test antenna is no greater than one meter, the far field of the two-meter-long test set-up is clearly well beyond one meter. It is not enough for the transmitting source to be in the far field of the receive antenna; the receive antenna must also be in the far field of the source transmitter. Now it could certainly be the case that the transmitting source at microwave frequencies happened to be a small slot or aperture in the test sample enclosure, and if that were the sole radiating structure, it could be a far-field measurement. But the point is, one cannot know that a priori.
Conclusion
Converting near-field radiated emission control from field intensity to antenna-induced limits brings the following advantages:
- Clear delineation between near-field vehicle and home/office/industrial plant far-field radiated emission controls;
- Better correlation between equipment-level radiated emission limits and system-level EMC goals (direct correlation with spectrum analyzer noise floor surveys of platform antenna-connected receivers);
- Diminution of wrong-headed EMC “engineering” comparing RE and RS controls as complementary functions; and
- As a corollary, it would raise awareness of the real issues involved in RE control and improve the quality of EMC engineering, reducing episodes where bad science is used to justify programmatic decisions.
Even if a majority of the EMC discipline found these arguments persuasive, it would be wildly unrealistic to expect that over a half-century of tradition would be overturned. Given that reality, but with the understanding that antenna-induced is the ideal for one-meter radiated emission control, significant benefits could still result. The major such benefit would be dropping SAE ARP-958 altogether and using polarization-independent far-field antenna factors. Another possible advantage would be the adoption of EMI test antennas that better modeled those connected to platform-installed receivers that are the motivation for the levying of radiated emission controls.
And whether or not we return to antenna-induced near-field limits, the discipline would benefit immensely if EMC engineers rediscovered the lost science of near-field measurements. They could do worse than reading NADC-EL-5515. It also might not hurt to read Hertz’s original work on his dipole.
A WWII historian once remarked that other historians read books previous historians wrote, and then write a new book based on what they had read. In contrast, this historian always cited WWII-era sources. There is something to be said for original sources. The Museum of EMC Antiquities exists to preserve such knowledge and foster its study until such time that the present Dark Ages give way to an EMC Renaissance.
Endnotes
- Pearlston, C.B. Jr., “The Systems Approach in Designing a Specification for the Control of Radio Interference in an Airborne Environment,” Proceedings of the 5th Conference on Radio Interference Reduction and Electronic Compatibility. October 1959. In this work, Pearlston compares RE limits for protecting radio reception to susceptibility limits simulating rf transmitters, and while acknowledging the true purposes of each type of control, still manages to compare such limits and conclude that “100 dB” margins exist.
- A complete derivation, leaving no “exercises for the reader,” is presented in Adamczyk, Bogdan, Foundations of Electromagnetic Compatibility with Practical Applications, John Wiley & Sons, 2017.
- Javor, K. “Journey To The Center of The Dipole,” In Compliance Magazine, September 2023.
- Javor, K., “On the Nature and Use of the 1.04 m Electric Field Probe,” ITEM 2011.
- MIL-STD-461, Electromagnetic Interference Characteristics, Requirements for Equipment, 31 July 1967.
- SAE ARP-958A, Electromagnetic Interference Measurement Antennas; Standard Calibration Method, November 1992.
- White, Donald R. J., “EMI Test Instrumentation and Systems,” Volume 4, Section 3.3.4 of the Handbook Series on EMI & EMC, 1971.
- Pearlston, C.B. Jr., Air Force Report No. SSD-TR-67-127, dated 1967. “Historical Analysis of Electromagnetic Interference Limits.” Pearlston makes the exact same points back at the inception that were presented in this article; these observations are hardly new:
“An academic argument could be made, and often has been, that “field intensity” is not a proper term to use in describing the phenomenon being measured and that “antenna-induced voltage” is much more descriptive of that phenomenon. Field intensity is generally defined as a measure of the intensity of the electric field; the term implies that the measured electric field gives a valid indication of the power density in the wave front, and permits an estimate to be made of the power coupled into a receiving antenna. Such an estimate is valid only in the far field where the plane wave phase relationships of electric and magnetic fields are fixed. Thus, the measurement does not give a valid indication of power coupling.
“Field intensity is also defined as the voltage induced in a conductor one meter long when held so that it lies in the direction of the electric field and at right angles to the direction of propagation and to the direction of the magnetic field. It can be argued that the equipment near-field does not have a uniphase front, and so the straight rod or dipole antenna will not necessarily be in the direction of the electric field. These near-field effects become even more marked at the higher frequencies where horn and parabolic reflectors are used.
“The term field intensity should refer to a phenomenon which is independent of the measuring instrument rather than, as in the present case, being so highly dependent upon the particular antenna used. The phenomenon measured is not the actual electric field of the wave front, but consists of indications of partial components of that wave front in the near-field of the test sample. A better name for the phenomenon would be “apparent field intensity”, but, as long as there is no confusion as to what is being measured, the name given to the phenomenon is not of great importance.”
Except of course his prognostication that the name change from “field intensity” to “apparent field intensity” is not really important was proven wrong, and in this case at least, Shakespeare may be more accurately paraphrased by saying that “A rose by any other name would stink.”
Part 1 | Part 2 | Part 3 |