On October 10, 2014, at about 1300 UTC, a Boeing 787-824 operated by United Airlines left London, bound for Houston. After about six minutes into the flight, the aircraft was struck by lightning. Three of five primary display units went blank, a status message related to a power distribution channel was displayed, and the captain’s forward windshield heater became inoperative [1,2].
The National Transportation Safety Board determined the probable cause(s) of this incident to be:
“A shutdown of the displays due to the inability to operate through a rapid transient radiated electric field brought on by a near field lightning strike. Contributing to the loss of displays for the remainder of the flight was the lack of guidance to the crew to perform a controlled power reset to the display.” [1]
This incident is significant because the cause of the display malfunction was not explainable in terms of the normal U.S. Federal Aviation Administration (FAA) lightning certification requirements and verification approaches. The display unit malfunctions could not be reproduced using appropriate testing protocols detailed in DO-160, Environmental Conditions and Test Procedures for Airborne Equipment.
Because of this, one needs to determine if a new E-field external environment requirement and a new equipment test method (per DO-160, Chapter 22) are required for civil aircraft certification.
That is the subject of this article, which is divided into two parts. The first part provides a summary of the main points from the report by the U.S. National Transportation Safety Board (NTSB) [2] for the convenience of the reader. The second part consists of explanatory material needed to go forward with defining a possible new external environment and equipment test method.
In the 1980s and 1990s, we were involved in several activities related to E-field effects for certification purposes. These included the near field lightning environment in MIL-STD-464, the in-flight data from the F-106 Thunderstorm research program, and research into the so-called “shock excitation” method for full aircraft testing. None of these resulted in an E-field certification standard environment or test requirements, and this subject has been dormant until now.
The conclusion is that both E and H fields play a role in the coupling of lightning energy into aircraft electronic systems, and a fresh look at how lightning E and H fields should be considered for certification is required.
Part I: Discussion of the B787 Lightning Event [2]
Event Description
The pilots of the fated 787 noticed that the three flight displays were not functional. However, there was no checklist item for a partial loss of displays, so the crew elected to use the checklist for “Loss of All Displays.” The procedure did not recover use of any of the three failed displays. The pilots found that window heat could not be restored. The remaining two displays were reconfigured, and the flight crew landed the airplane uneventfully at London’s Heathrow Airport (LHR).
After landing, LHR maintenance performed a lightning inspection and found external nonstructural damage in five locations along the nose. A power reset to the aircraft per maintenance manual procedures was accomplished, and the displays and other effected systems returned to normal function. After a review by United maintenance personnel, the aircraft was approved for dispatch and continued the flight to Houston with no further faults noted. Figure 1 shows the location of lightning attach points and the three affected displays.
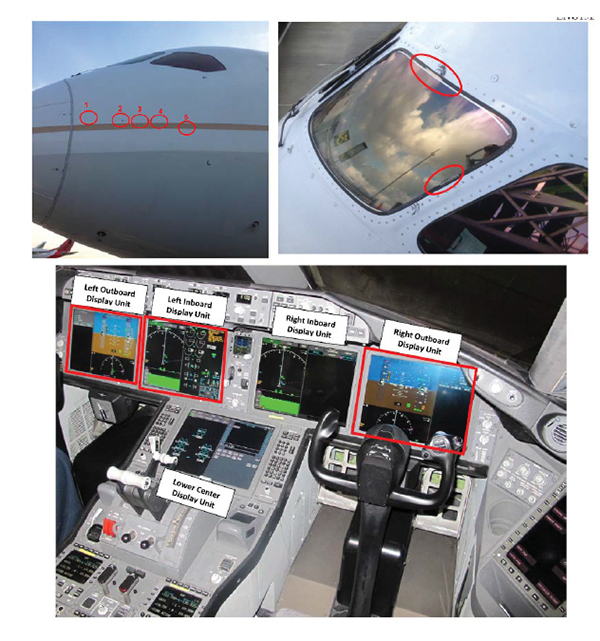
Boeing and Rockwell Collins (RC, who manufactured the displays) performed many tests and numerical computational electromagnetic (CEM) simulations to understand the event. The main conclusions were:
- The Boeing 787 aircraft was inspected and was found to be 100 percent in conformance with its original lightning protection design and certification. No exceptions were found;
- Similarly, the display units were tested to demonstrate their conformance to the appropriate DO-160, Chapter 22 certification test requirements. No exceptions were noted;
- Boeing and RC noted that the lightning indirect effects certification environments did not include a specification of the lightning-induced electric field. They then performed testing and analysis to determine if this could be the reason for the display blanking, error messages, and the loss of the windshield heat; and
- Their testing and analysis showed that lightning induced electric fields could indeed explain the event.
Boeing and RC Electric Field Testing
Test Approaches
Boeing and RC performed extensive E-field testing of the display units. The test articles included display units (DUs) from the event aircraft, and an exemplar (identical copy) DU.
The electric field environments were achieved in various ways:
- The MIL-STD-464 [3] near field environment from a lightning strike 10 m away from the test object:
- H Field: 2.2 x 109 A/m/s
- E-field: 6.8 x 1011 V/m/s = 680 kV/m/µs
- Discharging of charged parallel flat plates that enclose the DU
MIL-STD 464C E-Field Testing
Boeing and RC performed a MIL-STD-464 [3] near strike lightning test on an exemplar display and two displays from the incident aircraft. The test configuration is shown in Figure 2. The test setup utilized a Marx generator and a chopping ball gap. The DU under test was placed in between two parallel metal plates 1 m apart.
The testing resulted in DU blanking and generation of error messages. This would be for a presumed E-field rate of change of about 680 kV/m/µs.
E-field Lightning Testing by Discharging Charged Metal Plates
In the MIL-STD 464C testing, the DU was placed between two metal plates. The plates were charged to a certain voltage with a Marx generator, and this voltage was limited by the ball gap which discharged the plates, creating a large dE/dt (value of dE/dt not provided, but presumed to be at least 680 kV/m/µs). We note that this discharge current also creates dI/dt and dH/dt caused by current flow in the ball gap and the conductors leading to it.
For the testing described in this section, the parallel plates were charged by other voltage generators, such as applying static charge to the plates with an ESD gun. Once the plates are charged to a certain voltage, they are discharged with a shorting strap. The NTSB report does not provide the E-fields or the values of dE/dt provided by this testing, but presumably they are in the range of the MIL-STD-464C requirement or larger.
Figure 3 shows some of their configurations.
The discharge current path in this testing appears to be near the edge of the parallel plates, so magnetic fields dH/dt from the nearby current path also expose the DU. This point is further discussed below.
In general, their test results include blanking of the display and generation of error messages. An example of their results is provided in Table 1.
Test No | Approximate Charge Level | Charge Method | Test Plate | Discharge Point | Result |
1 | 7.0 kV | Injeco | Full Plate | LR | LCD Supply Voltage Fault |
2 | 5.0 kV | Injeco | Full Plate | LR | Grayscale Voltage Fault |
3 | 5.0 kV | HVPS | Full Plate | UL | LCD Supply Voltage Fault |
4 | 4.5 kV | HVPS | Full Plate | LR | LCD Supply Voltage Fault |
5 | 1.0 kV | HVPS | Full Plate | LR | No Blanking |
6 | 2.5 kV | HVPS | Full Plate | LR | Grayscale Voltage Fault |
7 | 4.5 kV | HVPS | Full Plate | LR | Grayscale Voltage Fault |
8 | 4.5 kV | HVPS | Strip Over Lower Edge | LR | LCD Supply Voltage Fault |
9 | 2.5 kV | HVPS | Strip Over Lower Edge | LR | Flickering |
10 | 4.5 kV | HVPS | Strip Over Lower Edge | LR | LCD Supply Voltage Fault |
Table 1: Example parallel plate test results
Boeing Aircraft Electric Field Analysis
Boeing also performed a CEM analysis of the electric fields present in the cockpit during a lightning event. This presumably was to determine any correlation between the experimental results and the expected vales of electric fields in the cockpit from lightning strikes to the event aircraft attach points.
Analysis also checked to see if the spark gap devices located in the window heater protection unit (WHPU) would activate during a lightning event (the spark gap did activate during the actual event). The analysis used three current waveforms (A, D and H) from SAE ARP 5412. Figure 4 shows some of the model features.
Example results are shown in Table 2, which shows E-fields greater than 1000 kV/m/µs. This can be compared with display susceptibilities of about 680 kV/m/µs during the MIL-STD-464 E-field test.
Case | Drive | Capt Outboard DU | Capt Inboard DU | ISFD | FO Inboard DU | FO Outboard DU |
Baseline 787 | A | 1800/3500 | 1040/1400 | 900/980 | 940/1600 | 920/1100 |
Baseline 787 | D | 1800/3400 | 1000/1300 | 950/1250 | 930/1300 | 900/1100 |
Baseline 787 | H | 2800/5000 | 1800/2600 | 1400/1700 | 1600/2100 | 2400/4900 |
Table 2: Example computational results: display E-field (average/maximum) peak derivatives (kV/m/µs)
The results showed that, based on the lower blanking thresholds demonstrated during the near strike lightning testing and the flat plate testing, the computed E-fields on the DUs exceeded the lower bound estimate of the display blanking threshold during every case where a “fast” spark gap (activated within 0.5 ns) fired or a waveform H was utilized.
Items Missing from the NTSB Report
Background
The NTSB report was somewhat incomplete in terms of the desire of the reader to know more information. These deficiencies are described in the following sections.
Separation of E-Field and H-Field Coupling Effects
The E-field testing fundamentally involved the discharge of charged metal plates that surrounded the DU. This discharge rapidly reduces the E-field to zero, creating a large dE/dt. At the same time however, the discharge current is likewise rapid, creating a large dI/dt as well as a large dH/dt near the current discharge path. The quantification and separation of these two coupling sources and their effects on DU electronics was not presented in the NTSB report.
For the MIL-STD-464 testing, the discharge path was the chopping ball, which does appear to be some distance away from the DU. Even so, the discharge current flowing from the edge of the plates to the ball gap provide a nearby dH/dt exposure of the DU. However, for the other parallel plate tests, the discharge current path appears to be immediately adjacent to the DU.
As an example, consider Figure 16 of the NTSB report, which is analyzed in Figure 5. This shows the discharge current for one of the DU parallel plate test shots. The peak current level is 491 A. The magnetic field 0.1 m (presumably in the location of the DU) from the discharge path is shown to be a factor of 68 larger than the MIL-STD-464 dH/dt. This brings up the question whether dE/dt, dH/dt or a combination, is the cause of the DU blanking.
A related observation can be made regarding the relationship of the spark gap activation and DU susceptibility. The CEM computations showed that the cockpit E-fields were large enough to cause DU susceptibility in two cases:
- When lightning Waveform H was the source; and
- When the computed coupling was large enough to activate the spark gap in the window heat circuit.
This brings up two questions:
- Is a new dE/dt environment really needed, if the effects can be caused by Waveform H? and
- The firing of the spark gap creates both a large dE/dt and a large dH/dt. Which is the real cause?
The second point is described with the help of Figure 6, which shows a simplified view of the cable (above the aircraft structure ground plane) and its attachment to the heater box. An external lightning source causes sufficient electromagnetic coupling to the cable so that the spark gap activates.
We note that when the spark gap activates, two things happen:
- The gap breakdown causes large dE/dt; and
- The gap breakdown causes large dI/dt with a large dH/dt
The NTSB report does not discuss the relationships of these two effects.
Display Unit Certification Method
The displays are presumably of criticality “Level A Display.” This means that the aircraft manufacturer has at least two options regarding the certification method:
- Level A Display certification can use the same technical approach used for Level A Control. This involves either full aircraft testing or full aircraft CEM simulations performed with a validated CEM model; or
- Level A Display certification can also use DO-160 equipment test waveforms selected by the installation features, according to the procedures in AC 20-136B and DO-160, Chapter 22. No aircraft test or analysis is required.
Any new certification approach used may depend on the details of any new E-field environment and equipment test method.
Summary of the NTSB Report
The key findings of the NTSB report can be summarized as follows:
- The event aircraft and DUs were correctly certified to IEL requirements;
- No exceptions were found in the maintenance history, installation, and grounding and bonding configurations of the event aircraft and equipment;
- Current industry standards for lightning tests do not include test conditions simulating a rapid transient radiated electric field;
- Boeing and Rockwell Collins testing demonstrated DU susceptibility as low as 680 kV/m/µs from the MIL-STD-464 E-field test requirement (MIL‑STD-464 is not required for civil aircraft). (Later, Boeing says the DU susceptibility was on the order of between 1000 kV/m/µs and 30,000 kV/m/µs). It is not clear how this relates to their MIL‑STD-464 testing);
- Boeing CEM analysis shows E-fields on cockpit DUs exceeding 1000 kV/m/µs;
- A strike by a waveform H can create E-fields large enough for upset on the DUs; and
- The CEM computations showed that the cockpit E-fields were large enough to cause DU susceptibility in two cases:
- When lightning Waveform H was the source; and
- When the computed coupling was large enough to activate the spark gap in the window heat circuit.
Part II: Interaction of Lightning Induced E-Fields on Aircraft
Background
We now turn to what is known about lightning related E-fields on aircraft. Information sources considered include:
- In-flight measurements of lightning striking the NASA F-106 thunderstorm research aircraft;
- Computations of E and H field coupling into apertures; and
- SAE ARP 5412 lightning direct effects E-field requirements.
NASA F-106 Measurements
The best lightning-induced electromagnetic fields on aircraft are found in three inflight research programs conducted in the 1980s:
- NASA F-106 Thunderstorm Research Program
- FAA/USAF Convair 580 research program
- The French Transall C-160 research program
Because the author of this article was intimately involved in the F-106 program, we refer to this data in our discussion.
The F-106 was instrumented to record electromagnetic fields and currents on the aircraft struck by lightning in flight. Approximately 700 strikes were encountered during the years 1982-1990. Three figures of the aircraft are shown in Figure 7. The results from this program have been summarized in two papers [4,5].
In Reference 5, there is a summary of measured surface displacement currents (= εo dE/dt), which is shown in Figure 8. This can be converted into the units used in the NTSB report with the relationship 100 A/m2 = 11,200 kV/m/µs ~10,000 kV/m/µs in round numbers.
Also in Figure 8 is a chart from the Boeing CEM results for cockpit fields, which are between 900 and 5000 kV/m/µs.
We see that surface E-field derivatives measured by the F-106 in flight are of the same order of magnitude, or sometimes much larger, than the cockpit computed fields.
The statistical distribution of the displacement currents on the F-106 surface is given in Figure 9.
Comparison of the Derivatives of External E- and H-Fields on the F-106
In day-to-day E3/EMC engineering, we commonly refer to a field as an E-field, and another as an H-field. We also commonly speak of E-field shielding and H-field shielding. But if an electromagnetic field varies in time and space, it contains both E- and H-fields.
Our implicit reference point is the plane wave, for which E/H=377 Ω in free space.
When we refer to a field as an electric field (E-field), we usually mean that E/H>>> 377 Ω (e.g., fields close to a dipole antenna). When we refer to a magnetic field, we usually mean that E/H<<< 377 Ω (e.g., fields close to a loop antenna).
The purpose of this section is to present the ratios of the E/H, or more specifically the ratio (dE/dt)/(dH/dt) on the F-106. These ratios have been previously published by the author [5, 6].
The evolution of E- and H-fields on aircraft struck by lightning is a complex process. Just prior to attachment, one expects that the electric field is large, and discharges from the aircraft will rapidly collapse the electric field, causing a large dE/dt. During this process, the magnetic field may be relatively small. Later on, however, for a return stroke flowing through the aircraft, the magnetic field derivative may dominate. Therefore, one may expect the ratio E/H and their temporal derivatives to be large early in the event, and to be smaller later.
To quantify E/H, we appeal to the F-106 research program [4,5], and we consider the transient digitizer data for the fast part of the events. The data is somewhat limited in application because the E- and H-field sensors are not located at the same place. However, if we consider the D-dot forward sensor on the fuselage under the nose and a tangential magnetic field derivative sensor on the fuselage near the top of the right-wing root, one can estimate the ratios of the peak values of dE/dt to dH/dt. The result is that for the measurements evaluated, it was found that this ratio was on the order of 1000 Ω.
To quantify these ratios at the exact same observation point, we employed a numerical simulation of these events based upon the 3D finite difference method. The simulations assume attachment at the nose with a tail exit. We considered four sensor locations; underneath the nose, on the side of the fuselage above the right-wing root, the tail, and in the middle of the underside left wing. Measured lightning currents from two in-flight strikes were used as sources, as well as a source current consisting of a linear ramp to a constant current with a 500 ns rise time. The current sources are shown in Figure 10. These sources are very small compared to lightning current waveforms in SAE ARP 5412.
Typical results are shown in Figure 11, and the results are summarized in Table 3.
Figure 10: Lightning current sources used in CEM simulations
Figure 11: Ratios of E/H at the same location from the F-106 CEM simulations
Sensor Location | E/H (Ω) |
Forward Lower Fuselage | 350-700 |
Left Wing | 500-2700 |
Tail | 300-500 |
Upper Middle Fuselage | 300-1000 |
Table 3: Ratio of E/H derived from experimental and computational results for the F-106
A Comparison of Electric and Magnetic Field Coupling to Cables Behind Apertures
Electric and Magnetic Field Coupling to Cables Behind Apertures
We wish to compare the coupling of surface displacement currents (normal E-field derivatives) to surface current densities (surface tangential magnetic fields) for coupling to a cable behind an aperture. Figure 12 shows coupling of surface magnetic fields, and Figure 13 shows a similar figure for normal surface displacement currents.
We see that the surface current density that would flow across a shorted aperture creates an electric dipole in the aperture. This dipole induces voltage sources in series with the cable according to a transfer inductance Lt.
On the other hand, the displacement current density that would exist normal to a shorted aperture creates a magnetic dipole in the aperture. This dipole induces current sources in shunt with the cable with a parallel transfer capacitance Ct.
Comparing the Coupling of External E- and H-Fields through Apertures in a Perfectly Conducting Plane
In this section, we summarize a paper [6] that isolates and compares the relative coupling levels for external E- and H-fields through an aperture in a perfect electrical conductor (PEC) plane. We numerically set up 3D canonical problems that can only respond to E-or H-fields so that we can separate out the coupling modes (E or H).
The problems all assume an 18-inch square aperture in a perfectly conducting infinite ground plane. The source wave shape is a plane wave sin-squared pulse having a full width of 50 ns with a 1 V/m peak amplitude. Both open circuit voltages and short circuit currents on a 2 mm diameter wire are computed.
The paper considers 11 configurations, but in the interest of brevity only a few results are reported here:
- Symmetry of the source and coupling geometry can exclude either E or H, so either H-or E-field coupling can be dominant; and
- For problems in which the aperture has a cavity behind it:
- The largest Voc is 4.8 mV for case 6 (Figure 14), which only involves E-field coupling.
- The largest Isc is 0.027 ma for case 7 (Figure 15). For this case, the H field is the main driver.
The bottom line for this demonstration is that there are cases for which either E or H external to the apertures are the main drivers of Voc or Isc. This means that coupling computations or full aircraft testing should consider both external E-and H-field sources.
Full-wave 3D CEM coupling codes are universally used by aircraft manufacturers to compute induced voltages and currents on cables, but the required simulation sources are only current waveforms from SAE ARP 5412. These computations, or equivalent aircraft level testing, are required for certification of level A control systems. (The aircraft manufacturer has the option of certifying the Level A Display systems on the same basis as Level A Control systems, but this is not a requirement.) There is no external criteria E-field environment that can be used for coupling through aircraft apertures.
However, no computations or aircraft level testing is required to determine the DO-160, Chapter 22 test levels for Level A Display systems. The aircraft manufacturer can select DO-160 defined equipment transient design levels (ETDLs) based only on the features of the display installation. None of the test levels involve an electric field specification.
Lightning Direct Effects E-Field Criteria in SAE ARP 5412
Although there is not an E-field criteria environment for Indirect Effects certification, SAE ARP 5412 includes four criteria voltage waveforms that are used for lightning attachment and direct effects certification purposes. These are known as Voltage Waveforms A, B, C and D.
Voltage Waveform A (shown in Figure 16) is the one most relevant to our discussion. This waveform rises at a rate of 1000kV/µs (±50%) until its increase is interrupted by voltage breakdown of the intervening air gap, resulting in the puncture of, or flashover across, the object under test. At that time the voltage collapses to zero. The rate of voltage collapse or the decay time of the voltage if breakdown does not occur (open circuit voltage of a lightning voltage generator) is not specified.
We note that if we apply Waveform A across a 1 m gap, we have a dE/dt of 1000 kV/m/µs, which by itself is in the range of interest for display upset.
A Path to Determine a Certification E-Field Criteria Environment
Below are some ideas regarding the path to determine if a new environment is indeed needed, and how to determine what it should be:
- A large E-field environment probably occurs during the attachment phase, or maybe during the phase of small pulses (Waveform H, for example);
- Does an existing current waveform (e.g, H) provide enough internal E?
- Does the NTSB report show that an E-field environment is needed? (note Waveform H created enough E-fields in the cockpit to upset display);
- Note that Level A Display certification can use DO-160 waveforms selected by the installation features. Maybe the certification approach for a Level A Display needs to be changed.
- One should research available in-flight aircraft measurements;
- Computational electromagnetics can be used to effectively define in-flight E-and H-field environments for various lightning scenarios;
- Laboratory testing can be used as a companion to the CEM effort to define the E-field environment;
- The corona sheath surrounding a lightning channel can be several meters in radius, engulfing the cockpit area. This should be investigated;
- What should be the time duration of dE/dt?
- If needed, a new equipment test procedure needs to be added to DO-160, Chapter 22.
Conclusions
There are no E-field or H-field requirements for the certification of aircraft to lightning indirect effects (IEL). But both E- and H-fields are part of the IEL coupling process.
Existing IEL certification external aircraft environments include only current waveforms that attach to the aircraft. They do not specify any E-or H-field environments,
The equipment certification environments in DO‑160, Chapter 22 include only bulk harness current waveforms, except for the pin voltage damage test. The pin voltage test values, however, are not derived from any electric field. Therefore, equipment certification requirements do not specify any E-or H-field environments.
Presumably, when full aircraft testing or CEM modeling is accomplished, the measured or computed results include wire harness currents derived from both E-and H-field coupling from the applied currents.
The CEM approach has the correct distribution of all E- and H-fields for the applied currents, because the aircraft is modeled in an infinite free space.
However, the accepted test methods enclose the test aircraft in a return conductor system (RCS), and the electric fields on the aircraft depend entirely on the RCS geometry, lightning pulse frequency content, and the termination impedance of the aircraft/RCS transmission line. In practice, the E-fields on the surface of the aircraft are orders of magnitude smaller than those for an aircraft in free space. This test method is not representative of an aircraft in free space.
In the 1980s and into the 1990s, we were involved in experimental and computational activities related to including an attachment-like E-field into full aircraft testing. The approach uses the RCS concept, except the termination was a spark gap or crowbar switch that would allow the generator to charge up the aircraft to a large voltage which would then collapse the voltage to zero, creating a large dE/dt. After the switch closes, the large current phase would occur. The term “shock excitation” was often used to describe this approach, but it never resulted in a useful method. Some of the reasons for this are that a large expensive high voltage generator is required, and also there was no
dE/dt certification requirement.
To make matters worse, neither the acceptable CEM approach nor the testing approach considers the electric fields from attachment or sweeping. This is a non-linear process that is not considered for IEL certification according to existing best practices.
Finally, even though we are advocates for considering E-fields in the certification process, we are not convinced that the NTSB report provides enough information to demonstrate that E-fields alone provided the necessary environment that caused the display unit upsets. Their reported data does not eliminate the effects of H-fields.
For electromagnetic fields that are both spatially and temporally varying, both E and H are present, as shown in Figure 17. A fresh look at how lightning E-and H-fields should be considered for indirect effects certification is required.
References
- National Transportation Safety Board Aviation Incident Final Report https://app.ntsb.gov/pdfgenerator/ReportGeneratorFile.ashx?EventID=20141011X20926&AKey=1&RType=HTML&IType=IA
- National Transportation Safety Board Systems Group Chairman’s Factual Report https://dms.ntsb.gov/pubdms/search/document.cfm?docID=462244&docketID=61058&mkey=90240
- MIL-STD-464C, Electromagnetic Environmental Effects Requirements for Systems
- Felix L. Pitts, Bruce D. Fisher, Vladislav Mazur, Rodney A. Perala, Aircraft Jolts from Lightning Bolts, IEEE Spectrum, July 1988
- Felix L. Pitts, Larry D.. Lee, Rodney A. Perala, and Terence H. Rudolph, New Methods and Results for Quantification of Lightning’-Aircraft Electrodynamics, NASA Technical Paper 2737, June 1987
- Rod Perala and T H. Rudolph, “A Comparison of Lightning Induced Electric and Magnetic Field Coupling Through Apertures,” presented at 1994 International Aerospace and Ground Conference on Lightning and Static Electricity, Mannheim, Germany, May 24 – 27, 1994
Relevant Lightning Certification Documentation
- RTCA/DO-160G, Environmental Conditions and Test Procedures for Airborne Equipment
- Aerospace Recommended Practice (ARP) 4754, Guidelines for Development of Civil Aircraft
and Systems. - ARP 4761, Guidelines and Methods for Conducting the Safety Assessment Process on Civil Airborne Systems and Equipment.
- ARP 5412, Aircraft Lightning Environment and Related Test Waveforms.
- ARP 5414, Aircraft Lightning Zoning.
- ARP 5415, User’s Manual for Certification of Aircraft Electrical/Electronic Systems Against the Indirect Effects of Lightning.
- ARP 5416, Aircraft Lightning Test Methods.
- ARP 5577, Aircraft Lightning Direct Effects Certification.
- AC 20-136B “Certification of Aircraft Electrical/Electronic Systems for the Indirect Effects of Lightning.”