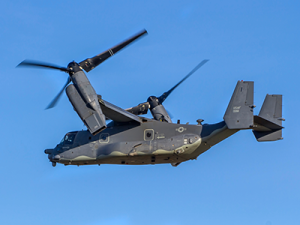
The Advantages of Addressing Lightning Related Issues Early On in a Design
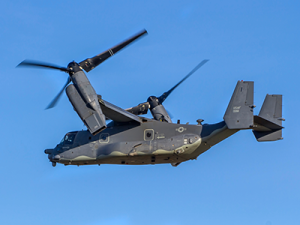
Even for traditional business and commercial aircraft, demonstrating compliance with U.S. Federal Aviation Administration (FAA) regulations pertaining to lightning can be challenging. From the prevention of ignition sources, to system functional upset testing, to the determination of actual transient levels, the process can be daunting. Couple this process with the advent of new and novel flight and vehicle concepts, and the task at hand can seem overwhelming.
In our 40 plus years of experience, we have seen in numerous companies that lightning is an afterthought with regards to new designs. Structural and flight considerations are generally given precedence, and lightning mitigation designs are applied over/around these concepts. On countless occasions, we’ve seen companies struggle with certifying a particular design feature simply because the design did not take lightning into account. Failure of certification testing can set programs back months, and these delays can be very costly.
The aviation industry as a whole has a number of design/protection measures that are commonly used, and these can alleviate many of the headaches associated with getting through the certification process. Non-conductive materials are commonly protected with diverter strips, composite materials typically contain some variant of expanded metal foil, surge suppression devices are used to keep lightning transients from overwhelming system components, etc. Additionally, the industry itself publishes a tremendous amount of information by way of Aerospace Recommended Practices to assist companies and manufacturers with getting their design in the air.
With the advent of new hybrid propulsion systems and short-range vertical take-off and landing (VTOL) aircraft, an even more challenging scenario approaches. With most traditional aircraft made of aluminum or composite materials, protection designs at a high level don’t differ significantly from one aircraft to another. However, in order for VTOL and hybrid propulsion vehicles to work efficiently, their designs are largely based around maintaining an extremely light-weight design.
This almost completely eliminates the viability of employing metallic structural elements. Metallic structures, while being extremely conductive, also provides a high degree of electromagnetic shielding. This shielding drastically reduces the transient levels seen on harnesses and system components inside the aircraft in comparison to those seen in non-metallic configurations/designs.
Especially in the cases of hybrid propulsion and short-range VTOL aircraft, the incorporation of metals and other conductive structures significantly increases the weight of the vehicle. Of course, this will reduce the total range of the vehicle and require more “energy” to operate the vehicle (thrust, etc.). All of these effects will inevitably increase costs, which runs counter to the competitive advantage offered by these modern vehicles (cost effective and short flight times). Thus, to create an aircraft design compatible with these operational criterion, many non-conductive and composite materials are used.
In our experience, most new companies focus their designs on meeting flight and operational requirements first (that is, meeting a specific market need or desire). Of course this is the preferred strategy for many because an aircraft that doesn’t fly isn’t particularly useful, and companies need to sell these vehicles to stay in business. The downfall with this approach is that, even if an aircraft can fly, it doesn’t mean it will be allowed to fly. If a particular design cannot demonstrate compliance to the applicable FAA regulations, it isn’t going to be of much use. In terms of complying with lightning-related FAA regulations, incorporating protection features late in the design process often forces significant changes across an entire aircraft.
In order to reduce the difficulty in demonstrating compliance to FAA lightning regulations, and all of the cost and schedule impacts as a result, a number of best-practice approaches can be used. We have worked on countless certification projects and have found these to be valuable starting points to build upon. These approaches provide significant cost and schedule savings if incorporated/utilized early in the design cycle. Many of these can be done later in the design cycle, but doing so increases the risk of certification challenges and increases the cost of incorporating them.
This task of protecting against the effects of lightning can be simplified however, even when dealing with the complex environments presented by hybrid propulsion and VTOL aircraft. This is done by leveraging our understanding of the behavior of the lightning channel by way of Maxwell’s equations and their consequences. We begin by segregating the effects of lightning into two groups, direct effects and indirect effects.
Direct Effects
The direct effects of lightning are defined as the physical damage effects at the points of lightning attachment. These effects can include melting, shock wave effects, temperature spikes, magnetic force effects, acoustic shock effects, ignition of flammable vapors, etc. These effects vary widely across materials, ranging from little to no effect to catastrophic failure.
With the development of new materials such as carbon composites, these effects are often far worse than originally expected due to the specific composition of the material. Carbon composites are made of many fibers that act like small conducting wires which causes the conductivity of carbon composites to be anisotropic, giving preference to currents parallel to the fiber direction. As the lightning channel dumps current into the material, the current densities are much higher than the fibers can handle, causing the fibers to vaporize. Combined with Lorentz forces, thermal expansion and acoustic shockwaves, the direct effects of lightning on carbon composites can lead to delamination, fracturing, holes and loss of material integrity. Figure 1 shows an example of these effects on a carbon composite panel.
In order to mitigate the direct effects of lightning attachment, several protective measures are employed. The protective measure used is dependent on both the material as well as the lightning attachment zone. Zones where attachment is unlikely require little to no added protection. However, for attachment zones or for areas in direct or close contact with those zones, careful consideration of the proper protective procedure must be made. Typically, expanded metal foils are used to increase the conductivity of the carbon fiber, where these foils are placed on or above the surface of the carbon but underneath protective dielectric coatings such as paint.
The first effect these foils have on the point of attachment is an improvement of the arc root dispersion, in which the attachment dwell time is reduced. This is due to the “bumpy” nature of the wire mesh which creates non-uniformities in the electric field near the surface (see Figure 2). This acts to divide the arc root into multiple current filaments instead of a single concentrated attachment. By dividing the lightning attachment into several filaments, the total current is divided between the several paths. This results in a decrease of the total dissipated energy by a factor of 1/n2, where n is the total number of current filaments. Because thermal and shockwave damage are dependent on the action associated with this energy, increasing the arc root dispersion greatly reduces these effects.
The second effect that these expanded metal foils exhibit on carbon fiber composites is due to the fact that the conductivity of the wire mesh is generally two orders of magnitude higher than carbon. This results in the lightning channel current flowing almost entirely within the foil, protecting the underlying carbon composites from high current effects. This does require that that the wire foil is well-connected to conductive structural components so that it is not simply a floating conductor.
For circumstances where the structure itself is non-conductive, breakdown and insulating capability are paramount. Two general approaches provide a way to minimize potential detrimental effects. The first approach is to provide enough dielectric insulation to ensure that the lightning channel does not puncture through the dielectric and attach to an internal conductive channel. This can be difficult to achieve in regions where lightning attachment is very likely and electric fields produced by internal conductors are high. Radomes are a prime example of this situation where high risk of lightning attachment and strong internal electric fields can result in puncture.
In order to further help prevent this issue in radomes, a second approach is also used whereby diverter strips are placed on the surface of the dielectric to help guide the lightning. This second approach can be further used to cause the lightning channel to fully “bypass” potentially risky areas and attach to a desirable conductor, known as a diverter. Because of the varying designs of VTOL aircraft and the trend of having very few conductive elements, this can be a challenging feat to ensure that the lightning attachment zones are well-protected and that the resulting currents are properly channeled through the airframe. The problem doesn’t stop there, however, since these resulting currents have their own effects on the aircraft.
Indirect Effects
Indirect effects are those effects associated with changing electromagnetic fields and structural voltage rises attributable to current flowing in the airframe. Electronic devices, especially solid-state electronics, are the most susceptible to these types of effects, which can range from short duration upsets to full component failure. As aircraft become more reliant upon electronic systems for flight-critical functions, the greater the risk of aircraft failure. Because VTOL aircraft often require electronic-systems for several flight-critical functions, they have a high risk of failure due to indirect effects.
The key to reducing indirect test levels is to work in tandem with direct effects protection to control where lightning currents flow through the airframe. In general, aircraft are encased almost entirely in aluminum or some other conductor which acts as a shield. For critical areas where the current needs to be guided or controlled, additional measures must be taken. This is done by diverter strips, extra shielding, strategic grounding and surge suppression devices. Because VTOL aircraft do not have the benefit of an enclosed conductive frame, the dangers of indirect effects are greatly magnified, requiring more robust protections than a conventional aircraft would need. For carbon composite designs, simply changing the orientation of the carbon fibers can have drastic effects on the current flow.
In order to quantify these indirect effects, actual transient levels have to be measured and then converted to a threat level that the system will experience. These types of tests are typically done via full vehicle and component testing, but this assumes that the entire design has been completed and built. If it is determined that there is a critical flaw in the design at this stage of development, it can be extremely costly. Furthermore, if a flaw is discovered through testing, the resulting damages can make the aircraft unusable and the point of failure difficult to identify.
Because VTOL aircraft have widely varying designs and functions that are far different than current certified aircraft, the lightning requirements and protections required are not straight forward and cannot be simply copied from existing solutions. Instead, it requires that each new design is carefully considered and tested to determine lightning attachment zones, resulting direct effects, and areas that will have high threat levels due to indirect effects.
In order to mitigate the costly approach of numerous tests on potential designs, simulations prior to full aircraft testing can be performed. These simulations include the modeling of the aircraft and building a full finite element simulation using Maxwell’s equations to obtain threat levels earlier in the design. This not only saves time and money for a given design, but it can also be used to simulate dozens of prototyped designs with different configurations with minimal cost increase. Once a given design has been adequately simulated with satisfactory results, it can then be built with far more confidence that it will pass certification testing.
To illustrate a possible situation where simulation can be helpful, we studied the anisotropic behavior of carbon composites using a full scale, simulated, prototype VTOL aircraft design. A single geometry was designed but the material properties of the resulting structure were parameterized to simulate the differences between aluminum and carbon composite (see Figures 3-5). This approach also provides the ability to adjust the directions of the anisotropic conductivity of the carbon composite. In these designs, the wings contain two aluminum supports and an aluminum spar connected to a rotating motor on the end of the wing allowing for horizontal and vertical flight modes. A simulated lightning strike on the tip of one of the carbon rotor tips results in currents flowing through the rotors, through the mounts, along the wing and down the fuselage, at which point the currents exit out the bottom of the aircraft.
For the aluminum shell, the currents stay on the outer wing of the aircraft with virtually no current flowing down the internal aluminum supports as shown in Figure 3. However, for the carbon composite structure, virtually all of the currents flow down the aluminum supports as shown in Figure 4 and Figure 5. The currents in these aluminum supports create changing magnetic fields which induce currents in any nearby wiring or electrical components. Any unshielded wiring within the wing would see currents high enough to damage connected electrical equipment, possibly even the motor itself. Because the outer skin of the wing no longer shields any internal wiring, separate shielding would have to be incorporated.
As shown in the above simulation, increased lightning protective measures will need to be made to accommodate new aircraft designs. To incorporate most of these necessary protections may require unanticipated increases in both weight and cost. VTOL aircraft generally have a small weight budget to begin with and, if these features are not incorporated into the preliminary design, it can easily make an otherwise efficient design inefficient or even unflyable.
In order to successfully design a VTOL aircraft, careful consideration of what lightning protection requirements need to be incorporated must be taken into account from the beginning. For example, long conductive wires connecting critical flight systems that go through or nearby lightning attachment zones need heavy shielding and protection. If those wires could be moved or the critical flight system moved to another location allowing wiring to be rerouted, this could easily offset the extra weight required for the change that would otherwise be needed for lightning protection.
Another possible solution would be to route wiring inside conductive supports which would control current flow and shield wiring at the same time. By looking at these requirements early in the design phase, poor design routes can be quickly eliminated, leading to a final design that will be far more efficient and safe than one that attempts to patch an existing design.
Moving Forward
Because the end goal is the safe transportation of humans and not simply a flying vehicle, VTOL designers need to carefully consider their project flow. While recent advancements in a flyable design may be exciting, the issues resulting from failure to certify the aircraft would be expensive at best and, at worst, could lead to the aircraft being grounded indefinitely. By having lightning in mind from the beginning, the hurdles can overcome systematically and efficiently, both in time and cost.
Using tried and proven simulation techniques is a key component in this strategy in order to conserve time and money. While it may require small sacrifices of time, money and weight up front, building the aircraft with safety in mind from the beginning can result in savings that far outweigh the alternative. Here are some important guidelines that can help facilitate the safe and efficient development of VTOL aircraft:
Develop Worst-Case Zoning—Because VTOL aircraft have a dual mode flight system, their lightning zoning will change depending on the mode of operation. However, because of their vastly varying designs, simply copying over the worst-case zoning from airplanes and rotorcraft is not sufficient. This is largely due to the number of rotors typically found on VTOL aircraft, their size and placement, and their need to rotate to accommodate the dual mode flight system. This is further complicated due to many wing design options between aircraft. Combined, these features present a unique type of aircraft that has no previous history of lightning certification. Because of this, each design must be carefully considered in each flight mode and then the worst-case zoning must be chosen for each zone. This requires an understanding of the aircraft and the lightning zone classifications, as well as the intentions behind lightning zone classifications as they apply to both aircraft and rotorcraft.
Consolidate Critical Components—Because shielding and protective measures are weight expensive, consolidating critical components in a single location enables the lightning protection to be consolidated as well. Shielding can be kept to a minimum, allowing the entire critical area to be shielded in order to help reduce indirect effects. This can help to reduce long cable runs which are susceptible to indirect effects. This approach also allows the lightning channel to be more easily diverted around the critical components.
Reinforce Lightning Attachment Zones—Because lightning strikes on carbon composites can cause material failure, it is important that all critical structural elements in lightning attachment zones are reinforced. This ensures that the direct effects of lightning attachment will not cause structural failure while still allowing the minimization of weight in areas where lightning attachment is unlikely. However, this approach relies on an accurate worst-case zoning approach as discussed above.
Simulate Early in the Design—By simulating lightning effects on a given design early in the design timeline, we can more easily determine if a given design tree will be effective. Because indirect effects rely upon induced magnetic fields, geometries, distances, material properties and current paths, simple intuition or clumped-circuit models cannot adequately predict potential threat levels. The wide variety of VTOL aircraft designs and their overall lack of shielding mean that simulation and testing be conducted. Because testing on prototyped designs is risky and expensive, simulations should be done first to determine problematic design features early on. This will allow necessary modifications to be made without the restrictions and complications imposed by late-stage design changes, saving both time and money in the process.