Our February 2024 column (refer to Reference 1, “Using a Near-Field Probe to Troubleshoot Transient Failures”) introduced a valuable technique for troubleshooting electric fast transient (EFT) failures at the PCB level. The same principle can be applied to troubleshooting radiated immunity failures. In this column, we present a case study to demonstrate these techniques.
In the following example, a company was caught by surprise when they discovered that a new IC daughterboard they designed and mounted on the original IC pinout location suffered from radiated immunity issues. The product, which is an audio device, exhibited tone distortion when exposed to radiated emissions in the 1.2 – 1.4 GHz range. The original IC had been in service for over ten years and was no longer available. The new IC, based on ARM architecture and powered by 3.3V, was intended to replace it.
The daughterboard (covering an area of approximately 374 mm2 and with a 5 mm distance to the main board) forms a capacitance of approximately 1 pF (calculated using the simple equation C=ε0εRA/h). The long trace from the daughterboard to the main board likely has an inductance of about 10nH. The self-resonance of this board is then estimated to be 1.5GHz, indicating a higher likelihood of immunity issues in this frequency range.
The immunity test was performed in an anechoic chamber using a far-field immunity test setup, but I only have access to a benchtop immunity setup, a challenge that many engineers face when their product fails the immunity test. They often wonder how they can troubleshoot the issue at their own bench. Additional questions arise, such as whether it is legal to perform a radiated immunity benchtop test and if there are health risks (they often ask, “Will I get cancer?”), associated with it.
Immunity Troubleshooting Using a Near-Field Probe
As shown in Figure 1, the approach we used involved connecting a near-field magnetic probe to a small RF power amplifier (assuming you have a signal generator to drive the amplifier). This setup can generate an intense field near the loop, exposing weak design points on the PCB to a strong magnetic field.
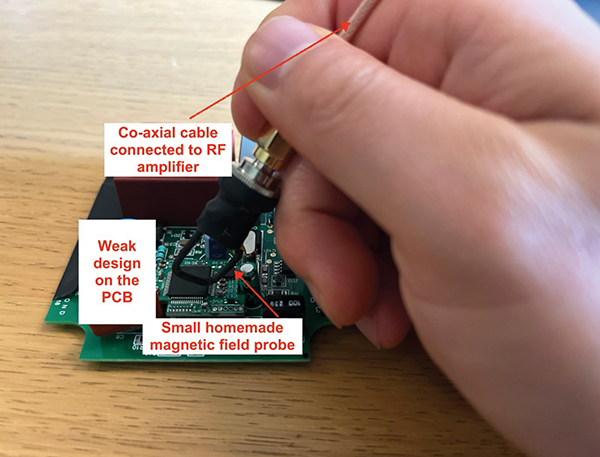
The detailed setup is shown in Figure 2. The idea was to replicate the same failure mode observed in the chamber, allowing us to troubleshoot and hopefully fix the issue on the bench.
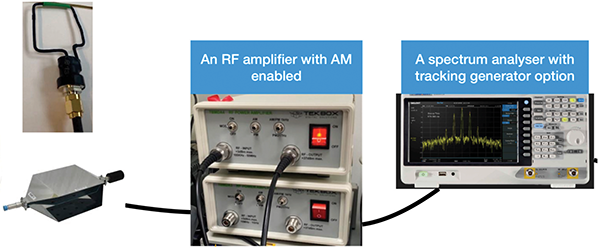
It’s important to note that during radiated immunity tests, noise can couple either through cables or directly via the PCB. In this case, we ruled out cable coupling because:
- At 1.2 GHz, a 2-meter-long cable typically cannot couple noise sufficiently (though exceptions exist, as always in EMC); and
- Common mode chokes were present on the cable connections.
In this case, when I held the near-field probe (connected to a Tekbox TBDA3B, which, at 1.2 GHz and 1.4 GHz, can still provide sufficient RF power despite being specified for use below 1 GHz) and moved it near the new IC daughterboard, the same failure mode occurred. I was using the AM modulation function on the amplifier, and the board produced a consistent rectified sound, which was very distinct.
Once the noise source was identified, it took some time to find a cost-effective fix, as the client is a small manufacturer with a budget constraint of less than one dollar. Eventually, we discovered that a small ferrite plate from Fair-Rite did the trick. After implementing this solution, the manufacturer tested the product in the chamber, and it passed the immunity test (for details, see Reference [2]). However, this situation highlighted a limitation of the technique.
The Limitations of Near-Field Probe Testing
When addressing the issue, I noticed that placing two or three ferrite plates between the motherboard and daughterboard showed improvement using my near-field probe. However, I couldn’t definitively tell the client whether two plates or three would guarantee passing the immunity test. In fact, I believed that even one plate might be sufficient, considering the intense field generated by my small near-field probe compared to the far-field conditions in the chamber.
As a result, I advised the client to test two samples—one with one plate and another with two. Ultimately, the product passed the test with just one plate, as I had suspected, but this couldn’t be conclusively determined from the near-field probe test alone.
When I was fixing the issue, I noticed that when I was applying two or three ferrite plates between the motherboard and daughterboard, I could see improvement using my near-field probe. But I was not able to tell my client whether two plates or three plates would be sufficient to pass the immunity test. In fact, in my opinion, even one plate would pass the test, considering the very intense field of my small near-field probe against what they tested in the far field in the chamber. So, I could only advise them to test two samples, one with one plate and one with two.
Eventually, they passed the test with one plate, as I would guess. But I could never tell from my near-field probe test.
Other considerations when using this approach:
- Magnetic field loops generally perform better than electric field loops.
- I used a homemade unshielded probe, but a commercially available shielded loop should work equally well. Generally speaking, you should avoid using pickup loops for injection tests (especially as described in [1]) due to the risk of voltage breakdown and heating in the probe. However, this should be fine for small RF signals.
- Given the small power applied to the loop and its non-directional characteristics, the risk of this test affecting nearby communication devices is generally very low.
- Most of the RF power will be reflected back to the amplifier rather than being consumed by the loop. So it’s advisable to place a 3- or 6-dB attenuator at the amplifier output (though most amplifiers are designed to handle this anyway).
- Another benefit of using magnetic field loops for this test is that you can use different loop sizes for different types of work. For small PCBs and high-frequency tasks, ensure your loop size is small enough. A rule of thumb I use is that the loop circumference should be smaller than half the wavelength at the frequency you’re troubleshooting.
- Finally, there is no substantial evidence to suggest that exposure to RF sources causes cancer. RF exposure (including thermal and non-thermal effects) is a separate subject, but using a small loop with a few watts output from an amplifier for short periods should be absolutely safe.
References
- Min Zhang, “Using a Near-Field Probe to Troubleshoot Transient Failures,” In Compliance Magazine, February 2024.
- Min Zhang, “Structure Resonances: Ways To Identify, Locate, and Fix EMI Issues,” Signal Integrity Journal, April 2022.