Near-field measurements are widely recognized as a highly accurate and versatile technique for testing antennas. The theory behind these measurements has been known for many decades. Indeed, in the 1960s, the company Scientific Atlanta marketed planar near-field systems where the Fourier transform operation was performed via operational amplifier circuits [1]. In the 1980s, spherical near-field measurements were introduced [2]. Since those days, especially in the past 25 years, these measurement techniques have become one of the preferred approaches for testing a broad range of antennas. Today, there are hundreds of near-field antenna test facilities installed across the globe, attesting to the method’s proven effectiveness and significance.
The acceptance of these methods and techniques was the driver behind the creation of the IEEE Standard 1720TM, “Recommended Practice for Near-Field Antenna Measurements” (IEEE Std 1720, see Figure 1.)
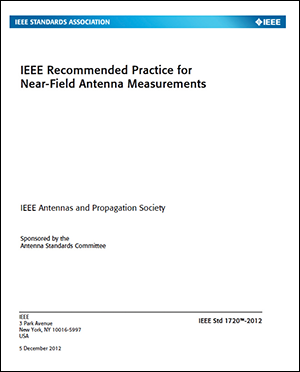
When it was initially approved in 2012, IEEE Std 1720 was a completely new standard developed by the IEEE Standards Association Standards Board (SASB). But advancements in technology and emerging developments over the past decade have made a revision necessary to ensure the document remains current. Further, the IEEE Standards Association (SA) mandates that all currently-active standards must be revised every ten years. For these reasons, the IEEE-SASB approved project authorization request (PAR) P1720 in 2019 for an SASB working group to undertake a revision of the original standard. To accomplish this task, a Working Group (WG) was formed under the Antennas and Propagation Society Standards Committee (APS/SC).
Currently comprised of approximately fifty committed volunteer members from industry, academia, and government, the WG is representative of the near-field measurement community, with both users and experts in the field. WG members hail from almost every continent, thus their work across numerous time zones has not been easy to manage. Nonetheless, the WG has been actively engaging in regular virtual meetings, with occasional face-to-face gatherings when possible. The primary focus of the WG has been on revising the existing material and identifying pertinent new NF measurement topics to be included in the updated standard.
To facilitate smooth collaboration, the IEEE-SASB has provided a dedicated workspace with an accessible database for all WG members. This comprehensive platform houses up-to-date documents and a complete history of developments [5]. Additionally, the workspace enables group decision-making through online discussions and electronic voting on various topics. The efficiency of this approach has significantly contributed to the progress of the WG’s efforts.
During the Antenna Measurements Techniques Association (AMTA) Symposium in 2023, a comprehensive report of progress on special topics was presented [6], which provided details on the proposed contents of the special topics section. In this article, we provide an update on the WG’s progress and highlight a few of the newer additions to the standard.
Content of the Revised IEEE Std 1720
As the current revision of IEEE Std 1720 is considered “minor,” the outline of the new document closely follows the original standard [3]. The main NF scanning geometries (planar, cylindrical, and spherical) are covered in detail. This original material is being reviewed and updated or rewritten depending on the level of review performed. The changes are intended to renew, update, and reflect widely accepted changes in technology and post-processing techniques. Here is the current draft outline of the revised Standard:
- Overview
- Normative reference
- Background
- Measurement systems
- Planar near-field scanning measurements
- Cylindrical near-field scanning measurements
- Spherical near-field scanning
- Non-regular scanning techniques
- Probes
- Determination of antenna gain
- Uncertainty analysis
- Special topics
Changes to the Main Clauses of IEEE Std 1720
The IEEE standard time convention for time-harmonic electromagnetic fields is of the form exp(+jωt), where “j” is the imaginary unit, “ω” is the the angular frequency, and “t” is time. Using this convention, the corresponding propagation phase factor is exp(-jkr), where “k” is the wave number and “r” is the propagation distance. This notation is sometimes referred to as the “engineering” time notation. This differs from the “physics” notation in which the “+” and “-” signs are interchanged in the above expressions. Throughout the standard, both time conventions are used without much distinction.
As the choice of convention does not matter as long as consistency is maintained, the WG decided to preserve the mix of engineering and physics time conventions in the Standard as foundational references exist using both conventions. Any new material based on commonly accepted practices will be in the engineering time convention. It is important that the convention used in the text is clear to the user of the standard.
In Clause 3 of the Standard, titled “Background,” the physics time convention is used predominantly. The rest of the document mainly uses the engineering convention. Mixed time conventions are commonly encountered in antenna measurements. It is particularly important that the system hardware/software implementations have the same convention to avoid erroneous results during near‑field to far-field (NFFF) transformation.
Clause 4 of the Standard is dedicated to the discussion of measurement systems used in near-field scanning. These systems require a combination of essential components, including a radio-frequency (RF) transmit and receive system, computerized scanning capabilities, data acquisition tools, and analysis software. The practical implementation of mechanical and electrical systems in these measurement setups can vary based on specific requirements, suitability, and the relative importance of various factors. The initial focus is on the acquisition of data on specific geometries, such as planes, cylinders, or spheres (see Figure 2). This provides practitioners with valuable insights into the selection of appropriate scanning systems that align with their specific measurement needs.
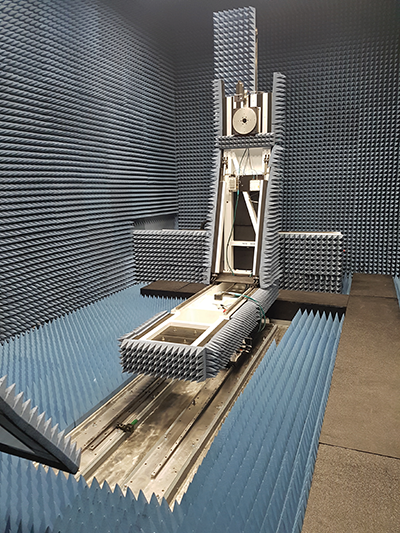
An important new addition to Clause 4 is a comprehensive discussion on modern anechoic chamber design, along with corresponding recommendations. This inclusion addresses the significance of optimizing the chamber environment for accurate and reliable near-field scanning measurements.
In the original 2012 version of the Standard [3], there was only a short paragraph on the RF absorber placement. Clause 5.3.1.8 of that version dealt with the absorber placement, but there were no specific recommendations for coverage except for planar near-field (PNF) scanning. Indeed, Clause 5 dealt with PNF scanning measurements. Hence, the only recommendation in Clause 5.3.1.8 of the original version of the standard was to treat the range surface in front of the antenna such that the main beam was maximally absorbed, thus reducing the possibility of a standing wave between the antenna and the end wall that could have cause errors on the measurement.
Much like was done with the IEEE STD 149‑2021 [4], the work presented in [7] on the updated version of IEEE Std 1720 is used to provide recommendations for the size and positioning of the RF absorber, not only for the PNF case but for the spherical and cylindrical scanning cases as well.
The recommendations presented aim to reduce the range multi-path to levels that are at least -40 dB lower than the direct path between the probe and antenna under test (AUT). In addition to these recommendations, the Standard points to references in the bibliography where optimizations of the absorber layout can be performed, as demonstrated in [8]. Figure 3 shows the possibility of using numerical methods to evaluate the illumination of the range walls to optimize the RF absorber coverage.
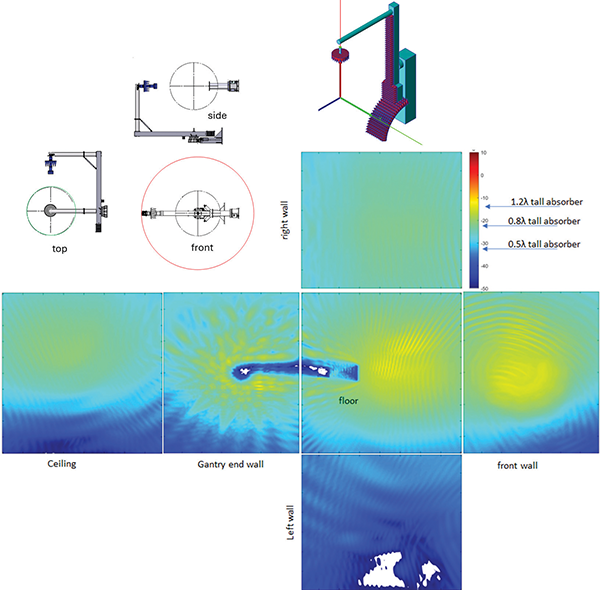
Clause 5 of the standard provides an overview of planar near-field theory along with practical implementation considerations. This method is particularly suitable for measuring antennas with moderate to high directivity. During planar near-field measurements, a probe is scanned over a planar surface located in front of the AUT. To transform the near‑field measurements into the far-field domain, a fast Fourier transformer is commonly employed. Planar near-field scanning is commonly employed for high directivity antennas due to the truncation of the scan area. The planar near-field scanning method was the first geometry for which probe correction theory was developed. The probe correction process is performed direction by direction, ensuring accurate and reliable results.
Clause 6 of the standard focuses on NFFF transformation techniques using cylindrical scanning. While this approach introduces a moderate increase in analytical and computational complexity compared to planar scanning, it offers the advantage of reconstructing the complete radiation pattern of the antenna, excluding the regions near the positive and negative cylindrical axes. In cylindrical scanning, the near-field data is acquired along a cylindrical grid and is thus particularly well-suited for fan-beam type antennas. By accounting for the effects of the probe, it becomes possible to accurately determine the far‑field pattern of the AUT. Also included in Clause 5 is a brief introduction to advanced scanning techniques aimed at reducing the number of measurement points (and thus measurement time) and the associated processing.
Clause 7 of the standard addresses spherical scanning techniques. It starts by providing a fundamental explanation of the theory behind spherical scanning, highlighting the use of probes with special symmetry properties, specifically the µ = ±1 probes. The benefits and advantages of employing these probes are thoroughly explained, emphasizing their significance in achieving accurate measurements. The clause has been further enhanced by an expanded discussion on higher-order probe compensation strategies. By incorporating probe compensation techniques of any order, practitioners can effectively minimize the impact of probe characteristics and enhance the accuracy of the measured results for a wider variety of probes. This comprehensive coverage of probe compensation techniques ensures that practitioners have the necessary tools and knowledge to perform precise spherical near-field scanning measurements.
Clause 8, titled “Non-regular scanning techniques,” encompasses the implementation of non-redundant sampling representations in different canonical scanning geometries such as planar, cylindrical, and spherical configurations. The primary objective of these techniques is to minimize measurement time. Furthermore, this clause also provides guidance on techniques applicable to the growing trend of sampling over non-canonical surfaces, highlighting the increased use of airborne drones and robotic systems for this purpose (see Figure 5).
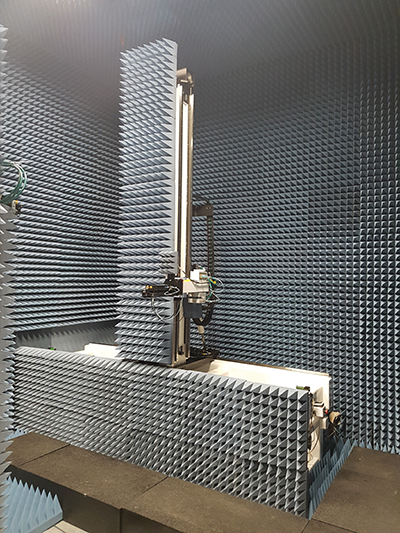
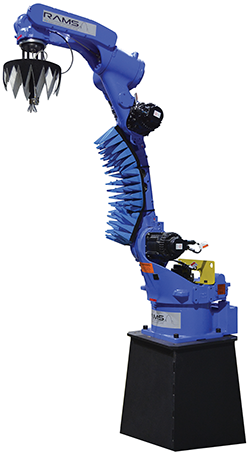
In Clause 9 of the standard, the selection and calibration of probes for near-field measurement applications are thoroughly discussed. The choice of suitable probes for near-field measurements is crucial as it directly affects the accuracy of the calculated far-field characteristics of the AUT. To ensure precise determination of the far field of an AUT using near-field data, it is essential to account for the probe’s influence during the measurement process. This necessitates knowledge of the probe’s on-axis gain and polarization characteristics, as well as its co‑polarization and cross-polarization patterns.
Clause 9 provides detailed instructions on measuring and determining these probe properties, enabling practitioners to accurately characterize the probe’s behavior. The clause offers guidance on selecting an appropriate probe for specific measurement scenarios. Factors such as the scan surface geometry and the desired measurement accuracy are taken into consideration to aid practitioners in making informed decisions regarding probe selection.
Clause 10 of the standard is dedicated to the analysis of gain in near-field measurement systems. Accurate gain determination techniques for near-field measurements are a fundamental challenge. The pursuit for precise gain measurements still revolves around selecting the most suitable gain measurement techniques for a given antenna and measurements scenario. Each technique inherently possesses its own limits in terms of accuracy, influenced by factors such as the measurement setup, environmental conditions, and the necessary equipment.
Additionally, the choice of technique can impact the efficiency and throughput of the measurement setup. Striking a balance between the financial investment required to obtain accurate gain measurements and the desired level of precision remains an ongoing challenge. This clause provides guidance on best practices to help choose the best methodology.
Clause 11 of the standard addresses the analysis of uncertainty in near-field antenna measurements. It serves as a resource for practitioners to understand and address the sources of uncertainty that can arise during the measurement process. The clause has been updated and revised to reflect recent changes and follows standardized procedures in line with widely recognized guidelines.
Clause 12 is dedicated to “special topics,” where various themes of relevance to near-field antenna measurements and post-processing techniques are described. Among these are several new techniques that are now widely accepted by near-field antenna measurement practitioners. [4]
Subclause 12.1 covers antenna system testing. This subclause has undergone some significant changes in the recent update. The term “antenna system” describes a device-under-test that consists of one or more passive radiating (or receiving) antennas that are connected to one or more active electronic devices and typically remain connected for the duration of the test. The testing of such systems typically aims at determining the receive
and/or transmit power performance parameters of the electronic devices connected to the antenna.
To illustrate what is addressed in this subclause, three example antenna systems are shown in Figure 6.
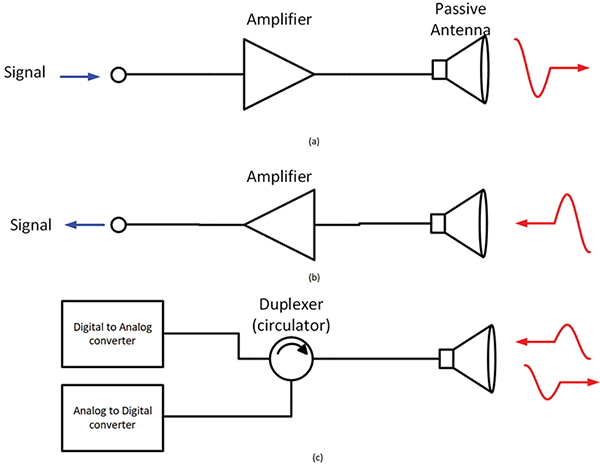
In configurations where it is difficult or undesirable to separate the active electronics from the passive antenna aperture(s), it is not possible to directly measure some component-level quantities of interest (e.g., aperture gain, transmitted power, receiver noise figure). However, it is possible to characterize combinations of these parameters that can be used to assess system-level performance.
This subclause of the standard describes methods for the measurement of common antenna system parameters of interest when the measurement probe is in the near field of the device under test. Subclause 12.1 provides recommendations on common methods of testing parameters such as equivalent isotropically radiated power (EIRP), saturating flux density (SFD), gain over noise temperature (G/T), effective isotropic sensitivity (EIS), and digital error rates.
Conclusion and Next Steps
The original 2012 version of IEEE Std 1720 expired in 2022 and is currently inactive. A Working Group of the APS/SC was formed to update the Standard, and this article provides an overview of the update and discusses the planned changes.
As of the time this article was prepared, the draft standard is on version P1720/D4. This draft has been reviewed by a small group of knowledgeable practitioners of the art of near-field measurements that have provided valuable comments for incorporation. In order to have time to complete these changes, the chairs of the WG have decided to file a one-year extension and go to balloting near the end of 2024, with the complete updated version of the standard slates for publication in early 2025.
Acknowledgment
The authors would like to recognize the hard work of the entire P1720 WG [5] for their continued dedication to the development of the standard.
References
- D. Hess, “Near-Field Measurement Experience at Scientific Atlanta,” Proceedings of the 1991 Symposium of the Antenna Measurement Techniques Association, Boulder, CO, October 7‑11, 1991, pp 5-3 to 5-8.
- J. E. Hansen (Editor), Spherical Near-Field Antenna Measurements, Peter Peregrinus Ltd., On behalf of the IEEE, London, UK 1988.
- “IEEE Recommended Practice for Near-Field Antenna Measurement,” in IEEE Std 1720-2012, December 5, 2012.
- “IEEE Recommended Practice for Antenna Measurements,” in IEEE Std 149-2021 (Revision of IEEE Std 149-1979), 18 Feb. 2022.
- https://ieee-sa.imeetcentral.com/p1720workinggroup
- L. J. Foged, V. Rodriguez, J. Fordham, J. Dobbins, and V. Monebhurrun, “Revision Progress: IEEE Std 1720 Recommended Practice for Near-Field Antenna Measurements,” 2023 Antenna Measurement Techniques Association Symposium, Renton, WA, USA, 2023, pp. 1-5, doi: 10.23919/AMTA58553.2023.10293439.
- Vince Rodriguez, Anechoic Range Design For Electromagnetic Measurements, Artech, 2019.
- M. Ingerson, and V. Rodriguez, “Recommendations for RF absorber treatment of ranges having a movable gantry or multiple probes,” 45th Annual Meeting & Symposium of the Antenna Measurement Techniques Association (AMTA 2023), Seattle, Washington, USA, October 8-13 2023.