Editor’s Note: The paper on which this article is based was originally presented at the 2019 IEEE International Symposium on Product Safety Engineering held in San Jose, CA in May 2019. It is reprinted here with the gracious permission of the IEEE. Copyright 2019, IEEE.
Introduction
Tin whiskers are small, typically hair-like structures that can form naturally from the surface of tin components in electronic devices (Figure 1). Tin whisker formation is a well-documented phenomenon that has been studied for decades. Throughout this time, little consensus has been reached regarding the particular mechanisms behind tin whisker formation. Whisker formation is believed to be a diffusion-controlled process motivated by stresses, both internal and external; however, the wide range of factors that may produce material stresses makes it exceedingly difficult to identify or isolate individual factors.
Previously, the use of lead-based alloys in electronic components and finishes mitigated the formation of tin whiskers. This protective mechanism was lost for many classes of devices with the passing of the Restriction of Hazardous Substances Directive 2002/95/EC by the European Union – followed by the adoption of similar restrictions in many other countries and regions – in which the requirement to use lead- free materials in electronic components and devices became mandatory. Tin-alloys offer a cost-effective alternative with high solderability and good corrosion resistance. The market choice to use tin-based alloys for solder connections and finishes has led to the reemergence of issues regarding tin whisker formation.
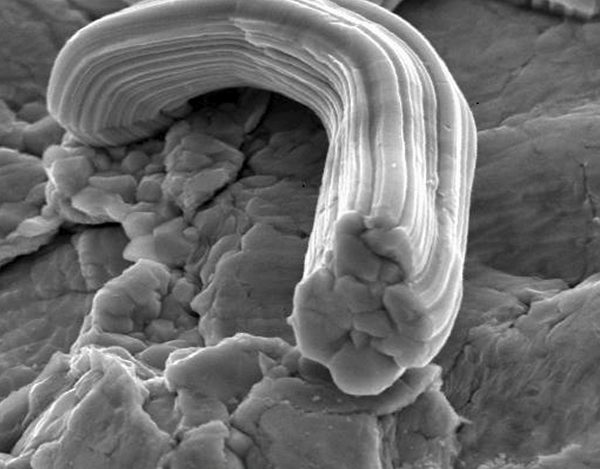
The consumer electronics market has grown exceedingly rapidly in recent decades, which has driven the development of faster and smaller electronics technologies. As the demand for faster processing and smaller packaging has increased, the vulnerability of highly compact semiconductor devices and shortened interconnect spaces has also increased. Electronics are embedded in countless devices across products and industries, and similarly countless automated systems depend on their precision and reliability. These competing drivers must be factored into a wide range of engineering considerations for the design and operation of modern electronic devices.
ADAS and Autonomous Driving Systems
Electronics failures in non-safety critical systems are inarguably a nuisance. In contrast, for safety-critical systems strategies must be in place for error handling and failure mitigation when a problem arises. These types of control systems are critical to the safe operation of systems in aviation, military applications, and the automotive industry. Even what the layperson thinks of as a traditional vehicle on the road today is dependent on a broad range of interwoven electronic systems. These systems include:
- Powertrain and chassis control
- Engine, transmission, hybrid control
- Steering, brake, suspension
- Integrated systems/services
- Multimedia (infotainment) applications
- Car audio, traffic information
- Body electronics
- Instrument panel, key, door, window, lighting
- Air bag, seat belt
Adoption of new driver assist technologies requires an extensive infrastructure building on those electronic systems listed above. Electronically controlled features of autonomous vehicles expand the above list to more sub-systems including, but not limited to:
- Parking assistance
- Lane departure warning and lane keeping assistance
- Electronic stability control
- Forward collision warning
- Automatic emergency braking
- Dynamic cruise control
- Car navigation and position sensing
- Vehicle-to-vehicle (V2V) and vehicle-to- infrastructure (V2I) communications
- Driver alertness monitoring
Many of the essential technologies supporting automotive sensors and electronic actuators used to navigate the complex environment of roads, vehicles, signs, and pedestrians in driver- assistance systems are not fundamentally new; however, the newly developing way in which these devices are used to decide, for example, how a vehicle should traverse an intersection has driven the need to increase the safety and reliability of these systems. Continuous innovation and evolution in system integration and packaging is crucial to achieving such advancement in embedded automotive electronics.
The utilization of components not originally intended for automotive applications has brought about new challenges as these systems are employed in the vastly different automotive environments of temperature and humidity extremes, sand and salt exposure, and vibration and shock, to name just a few. A relatively new entrant into the automotive industry is the use of LIDAR systems to image a vehicle’s surroundings, identify detected objects and infer how they might change or move a moment later. A LIDAR technology system that was previously developed for indoor, low-risk consumer electronics applications requires significantly less rigor in its design, documentation, and validation than a LIDAR being used for pedestrian detection in an autonomous vehicle. Every aspect of an electronic system such as this one in an autonomous vehicle is critical to ensuring passenger, pedestrian, and infrastructure safety.
The typical trend in consumer electronics is a steady push for miniaturization of components and increasingly compact packaging. The resulting evolutionary course has allowed for increasingly dense packages capable of higher and higher levels of performance. This trend has been fundamental to the rapid evolution and proliferation of electronics in seemingly all aspects of consumer products; however, the vast majority of these implementations are not designed with consideration for safety-critical operations, as by-and‑large
they are not intended for such applications. The design paradigms of consumer electronics cannot be applied in their traditional sense to safety- critical applications, as a number of enabling features of low- cost electronics run counter to safety-critical design requirements. High-density arrays of components and electrical circuit traces present multiple mechanisms by which faults may be induced, and packaging of multiple sub-systems into integrated systems may allow for unintended or unmanageable propagation of faults between sub-systems. In effect, as the design space of integrated systems broadens, so too do the potential effects of fault or failure.
With the removal of lead from tin solders and finishes, the importance of addressing and implementing mitigation strategies relating to tin whisker formation has become imperative. Many of the failure modes present in driver assistance-enabled vehicles are the same failure modes of systems in cars today. However, there is a growing trend of greater integration of automotive electronic systems and the diverse electronic components and sub-systems must be interoperable, not only with respect to electrical function, but from physical packaging and positioning requirements.
Development and implementation of a new technology requires extensive safety considerations within the product life cycle. These requirements can lead to unforeseen second and third order effects as the function—or failure—of one component may cascade in numerous ways. With the heavy reliance of autonomous vehicles on electronic systems, including driver assist systems currently available in vehicles on the road today, it is important to understand the implications of tin whisker formation on vehicle electronics.
Tin Whisker Formation and Implications
Metallic whiskers are electrically conductive structures that can form from a variety of metals, including gold, silver, zinc cadmium, indium, and antimony, but are most often associated with tin. Tin whiskers most often occur where tin has been used as a final surface finish, for example on surface mounted integrated circuit pins (Figure 2). These tin whisker structures have often observed to have lengths of several millimeters but can grow to lengths in excess of 10mm. [2]
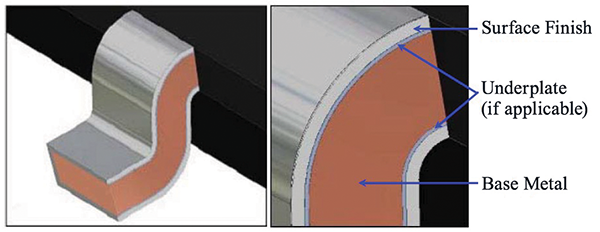
Incubation time for whiskers can be from days to years, meaning that long-term electronics applications are vulnerable to tin whisker growth. Scientists cannot accurately predict whisker formation other than to say whiskers are likely to form on pure tin. Tin whiskers can be hard to detect, especially because of their extremely small cross-sectional dimensions of approximately 1-10um (approximately one tenth the diameter of a human hair) (Figure 3). While these structures are small, due to their electrical conductivity, even microscopic tin whiskers can carry current for a short period of time.
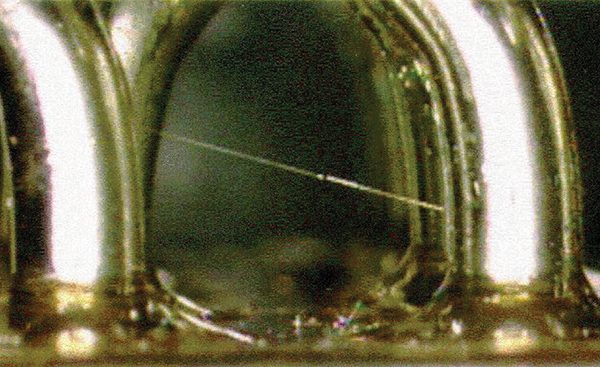
A wide range of factors are attributed, but not proven, to cause tin whisker growth, often believed to be a result of direct or indirect contribution to material compressive stresses or promotion of tin diffusion. [4] Factors that may, under certain circumstances, cause tin whisker growth include:
- Temperature
- Humidity
- Surface finish
- Internal stresses
- External stress
- Temperature change
- Vibration
- Lattice structure
- Lattice interstitials
- Magnetic field
- Voltage differential
- Altitude (ambient pressure)
- Manufacturing or process abnormalities or damage
This wide range of contributing factors presents a complex interplay of first, second, and higher-order effects that, to date, have not been fully mapped. It is quite possible that multiple individual factors in combination may be required to achieve whisker growth on a particular electronic component. It is important to note that studying tin whiskers with accelerated life tests has proven challenging because they do not appear to grow any faster or sooner in numerous simulated environments.[5] This implies that certain growth factors, or combinations of factors, may be so poorly understood as to not be properly represented in accelerated life tests. The present consensus in the literature is that conditions that increase the stress in a tin film or promote diffusion tend to induce whisker formation. [6], [7] For example, a mismatch in coefficients of thermal expansion between a tin surface finish and the base material can create stress in the finish as temperature varies. Conversely, stress can be generated from within the tin surface finish due to variability in the microstructure of the tin surface finish. The Joint Electron Device Engineering Council (JEDEC) notes that:
“[T]here is at present no way to quantitatively predict whisker lengths over long time periods based on the lengths measured in short-term tests… [T]he fundamental mechanisms of tin whisker growth are not fully understood and acceleration factors have not been established. Therefore, [accelerated aging testing] does not guarantee that whiskers will or will not grow under field life conditions” [3]
Induced Faults Resulting from Tin Whisker Formation
The formation of tin whiskers can have detrimental or catastrophic effects on electronic systems through a number of means. Tin whiskers may form across conductors or may break from tin structures and fall onto other conductors thereby forming a conductive bridge. In these scenarios, the conductive whisker may create an electrical connection where one was not intended. If these conductors are of similar voltage potential, then no short circuit current will flow. However, if the conductors are at differing potentials (i.e., between power and ground) then a short circuit current will flow but may be brief as the microscopic tin filament may be consumed by the event. Many electrical circuits in automotive electronics are current limited, and in the event that a tin whisker forms a short circuit across conductors in a current limited circuit, then the short circuit current that flows will be inherently limited by design. In other cases, intermittent short circuits may occur if the whisker is moved into and out of contact by vibration, air currents, or other means. In conditions involving both high currents and voltages, a whisker may be vaporized and form a vapor arc composed of metallic ions; such arcs may sustain very high currents and may cause extreme damage. [8]
Tin whiskers may also severely impact the performance of micro-electromechanical systems (MEMS) and optical devices as a physical contaminant. A tin whisker that grows or falls onto a MEMS structure can interact with that component’s operation by significantly altering mechanical properties (e.g., the mass of an accelerometer) and thus alter the overall performance of the device. Likewise, should a whisker become deposited on optical components, it may severely degrade performance, for example by physically obstructing the lens and therefore the operation of the entire device. Similarly, tin whisker formation on RF components used for communications may have detrimental effects on the functionality and performance of devices due to the tin whisker altering the frequency properties of an antenna used for either transmitting or receiving radio signals. Due to the high precision of many of these electronic and electro-mechanical devices, even subtle alterations that tin whiskers may have on the device’s physical and electrical properties should be considered.
Electronic system failure modes can range from anomalous output signals to catastrophic component failure, and these failure modes must be analyzed and understood such that adequate mitigation strategies can be employed. For example, an electrical short circuit across two pins of an automotive sensor connector due to tin whisker growth across the proximal conductive surfaces may result in an incorrect signal being sent to an associated control module. However, mitigation strategies that check and confirm that the sensor output is correct can act to trigger a diagnostic trouble code upon error detection and transition the vehicle into a safe operating mode.
Standards and Guidelines Related to Tin Whiskers
While the details of tin whisker formation are not fully understood, their detrimental effects are, and some standards have been developed to assess whisker growth and thereby manage the risk presented by whiskers. While these early standards may be limited in scope, it is expected that they will be further matured as understanding of whisker growth mechanisms become better understood.
IEC60068-82-2 – Environmental Testing Part 2-82: Whisker Test Methods for Electronic And Electronic Components provides an international standard for ambient environment, elevated temperature and humidity and temperature cycling of electronic components to determine potential for whisker growth. This standard also offers a maximum acceptable whisker length allowable in devices and components following test series. [9]
JESD22-A121A – Measuring Whisker Growth on Tin and Tin Alloy Surface Finishes details ambient storage, elevated temperature and humidity, and temperature cycling tests to be performed to as well as inspection processes for categorizing tin whisker density. This standard does not provide acceptance criteria. [1]
JESD201A – Environmental Acceptance Requirements for Tin Whisker Susceptibility of Tin and Tin Alloy Surface Finishes describes a methodology for environmental acceptance testing of tin-based surface finishes and mitigation practices for tin whiskers. The standard identifies classes of electronic devices and components based on criticality of their function and associated maximum allowable whisker length following JESD22-A121A testing. [3]
JP002 – Current Tin Whiskers Theory and Mitigation Practices Guidelines provides explanation of the theory behind tin whisker formation as of the publication date (03/2006) in addition to mitigation strategies to prevent tin whisker growth and efficacy analyses. [10]
GEIA-STD-0005-2 – Standard for Mitigating the Effects of Tin Whiskers in Aerospace and High-Performance Electronic Systems details control levels for tin avoidance and whisker mitigation and risk acceptance for each control level. These control levels are largely similar to those in JESD201. [11]
It is important to note that the testing proscribed in each of these standards is based on environmental conditions; specifically, elevated temperature and humidity and temperature cycling processes. While these conditions are often used for accelerated aging testing of various commercial products, there currently exists no correlation or quantifiable coefficient to directly relate these tests to operating conditions. Specifically, JESD201A elaborates that neither tin whisker growth nor length can be predicted because neither the mechanisms, nor acceleration factors, are as of yet understood. [12] While these standards may be used to gain a gross understanding of the potential for electronic devices or components to form tin whiskers, the current understanding of whisker formation limits the ability of any test series or standard to quantitatively determine the potential for, or severity of, whisker formation outside of analysis of in-service samples.
Design Considerations for Tin Whisker Mitigation
Because the mechanisms that promote tin whisker growth are largely undefined and poorly understood, the tools available to mitigate both the formation and impact of tin whiskers cover a broad range of design considerations and applications. It is important to understand that due to the enigmatic nature of tin whisker formation, there is no known singular remedy or inhibitor to whisker growth. For this reason, an understanding of the costs and benefits of each design aspect is critical, and trade-offs between cost and benefit may be highly dependent on specific design objectives and requirements. Furthermore, while some design considerations may be taken to inhibit tin whisker growth, there are also design practices that may be undertaken to mitigate the potentially detrimental impact of tin whiskers should they form. Even without a comprehensive understanding of the factors governing tin whisker formation strategies can implemented to handle potential system faults that may occur.
Design Considerations to Reduce Potential for Tin Whisker Growth
Tin whisker growth is correlated with a variety of factors that may occur in the design, fabrication and function of electronic components, MEMS, and optical devices. While no single consideration is assured to prevent tin whisker growth, implementation of one or more consideration may significantly reduce the potential for tin whisker formation. Some of the most readily implemented mitigating design considerations to prevent tin whisker growth are [13]:
- Use of lead/tin solder chemistries, avoid use of pure tin
- Avoidance of electroplated or “bright” tin finishes
- Increase grain size through addition of alloying elements
- Increase grain size through fabrication and manufacturing processes
- Inclusion of vibration isolation elements on circuit board mounting structures
- Separation of circuit board traces and connectors with high voltage differential
- Application of conformal coatings to board surfaces
- Reduction or relief of residual stresses
- Minimize introduction of stress through processing, assembly, and operation
- Maintain process and fabrication control to prevent surface damage or marks, and resulting stress concentration or loss of protective coating (Figure 4)
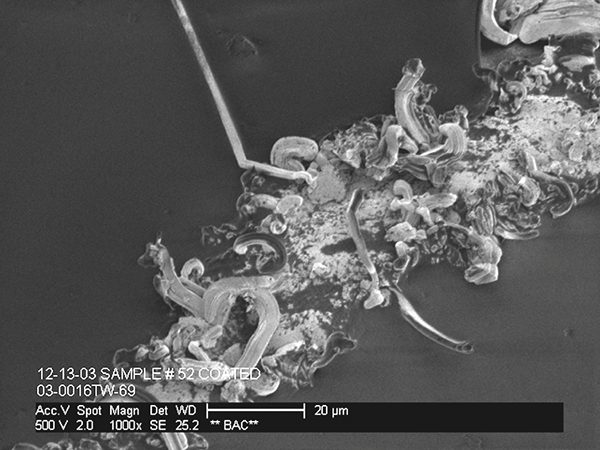
Whiskers have been observed to form under, and eventually penetrate protective coatings, allowing them to directly interact with other—supposedly isolated—components; [13] however it should be noted that conformal coatings offer two layers of protection between conductors, requiring two points of failure before a whisker can create such a failure. Even in cases where whisker formation does not result in direct electrical contacts, compromise of protective coatings may result in a path for further environmental damage to the underlying electronic components.
Design Considerations to Reduce Impact of Tin Whiskers
While efforts may be undertaken to reduce the potential for tin whisker growth, none of these are assured to fully prevent whisker formation. Further design considerations may be implemented to reduce the impact of tin whiskers should they form. As with whisker formation considerations, these may be used in combination as part of an overall design strategy. Commonly implemented design practices for mitigation of the impact of tin whisker formation include:
- Separation of board traces to reduce the potential of whiskers of sufficient length to bridge the gap
- Implementation of physical barriers to prevent shorts
- Application of conformal coatings, potting materials, or other means of encapsulation
- Shielding or segregation of MEMS and optical devices from electronic elements
Design Considerations for Functional Safety
Numerous efforts can be made to reduce tin whisker growth and to reduce the impact of tin whiskers if they grow; however, ultimately there must be fault handling and mitigation strategies designed into electrical and electronic systems.
Functional safety design concepts outline a method by which hazards and risk are first identified and then the tolerability of those identified risks is evaluated such that the necessary level of risk reduction can be determined. This is a process that is applied across components and systems. A set of safety requirements is defined for each risk reduction target and a safety integrity level (SIL) is assigned to each safety requirement. In every step of the safety life cycle, rigorous documentation is performed in order to catalog the process. Ultimately, a design then incorporates safety functions, which are the means by which the system meets its safety requirements, and extensive validation is performed on the implementation of these functions.
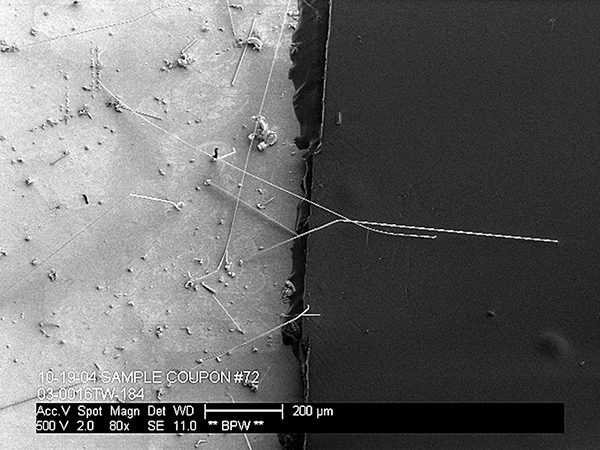
Conclusions
The propagation of electronic sub-systems and components in automotive systems represents an unprecedented intersection between safety-critical devices and consumer electronics. Never before have electronic devices played such a critical role in systems so ubiquitous. However, related standards – such as those surrounding a subject such as tin whiskers – have failed to fully appreciate the class into which these devices should fall and the associated repercussions of such classification.
Tin whiskers represent a threat to electronic systems that, at current, has poorly understood root causes. To date, no singular mechanism has been definitively proven to directly lead to whisker formation. While the presence of internal or external stresses has been attributed to whisker formation, the vast range of factors that can result in component stresses makes it nearly impossible to eliminate all such sources. While limited and only relatively recently developed, standards exist in industry to assess the potential for tin whisker formation, these standards are, at best, poorly correlated with real-world conditions and do not serve as reliable predictors of whisker formation in nominal operational conditions, as opposed to prescribed test conditions.
Due to the challenges in predicting or quantifying the potential for whisker growth, it becomes necessary to implement a holistic approach to prevention of whisker formation as well as mitigation of the impact that tin whisker formation may have on a system. A comprehensive approach will include design decisions to reduce the potential for, and rate of, whisker formation; mechanisms to mitigate the immediate effects of whisker formation; and system-wide design features to limit the potential damage caused by whiskers.
Implementation of these design considerations may exceed or diverge from existing standards, which at present may not be particularly relevant to assessing or predicting the risk of tin whiskers to a given system design. The design of complex and safety-critical systems should take particular note of the possible contributors to, and effects of, whisker formation and include provisions to protect against this danger.
References
- JEDEC Solid State Technology Association, JEDEC Standard JESD22-A121A Test Method For Measuring Whisker Growth on Tin and Tin Alloy Surface Finishes, Arlington, VA, 2008.
- NASA, “Basic Information on Tin Whiskers,” 16 June 2009. [Online]. Available: https://nepp.nasa.gov/whisker/background/index.htm. [Accessed 9 January 2019].
- JEDEC Solid State Technology Association, JEDEC Standard JESD201 Environmental Acceptance Requirements for Tin Whisker Susceptibility of Tin and Tin Alloy Surface Finishes, Arlington, VA: Reaffirmed May 2014, September 2008.
- King-Ning Tu, Jong-ook Suh, Albert Tzu-Chia Wu, Nobumichi Tamura, Chih-Hang Tung, “Mechanism and Prevention of Spontaneous Tin Whisker Growth,” Materials Transactions, vol. 46, no. 11, pp. 2301-2304, 2005.
- A. Gupta, “Hard Won Knowledge Mitigates Effects of Tin Whiskers,” Electronic Design, 2014.
- L. Panashchenko, Evaluation of Environmental Tests for Tin Whisker Assessment, March: MS Thesis, University of Maryland, 2009.
- M. Osterman, “Tin Whiskers Remain a Concern,” Surface Mount Technology, vol. 30, no. 2, pp. 50-54, 2015.
- JEDEC Solid State Technology Association, Current Tin Whiskers Theory and Mitigation Practices Guidelines, Guideline JP002: Reaffirmed September 2016, March 2006.
- IEC, “60068-2-82 Ed. 1 Environmental Testing – Part 2-82: Tests – Text TX: Whisker Test Methods for Electronic and Electric Components,” 2007.
- JEDED/IPC, “Current Tin Whiskers Theory and Mitigation Practices Guideline,” JP002, 2006.
- Government Electronics and Information Technology Association, “SEIA Standard GEIA-STD-0005-2,” 2011.
- Lavery, Patrick, Vicor Corporation, “Strategies to Mitigate The Tin Whisker Phenomenon,” [Online]. Available: http://www.vicorpower.com/documents/rohs/tin_whisker_mitigation_strategies.pdf. [Accessed 11 January 2019].
- Woodrow, Thomas A. and Ledbury, Eugene A., The Boeing Company, “Evaluation of Conformal Coatings as a Tin Whisker Mitigation Strategy,” in IPC/JEDEC 8th International Conference on Lead-Free Electronic Components and Assemblies, San Jose, 2005.