Product Safety Newsletter – May/June 1990
Recently, a colleague asked, “How do I select the fuse rating for a new product?” His cord-connected product has a switching-mode power supply and consumes 170 watts, with maximum normal-mode input current of 2.74 amperes. One source suggested a rule-of thumb that the fuse rating should be about 1.5 times the maximum normal mode input current. If he used this rule, he would use a 4.2-ampere fuse. On the other hand, he had another, similar product, also with a switching-mode power supply, consuming 180 watts. Its maximum normal-mode input current was 3.2 amperes, and it used a 3-ampere fuse! Naturally, with this gross difference between two similar products, my colleague was confused.
Let’s try to reduce the confusion.
I’m limiting my discussion to “small-dimension” fuses. Generally, small-dimension fuses are those that do not exceed 13/32-inch in diameter and 1-1/2 inches in length. Small-dimension fuses include the popular 5 x 20 millimeters and 1 /4 x 1-1 /4 inches dimensions. In the parlance of UL 198G, these fuses are “miniature” and “micro.”
Before you can select the fuse current rating, you must first answer the question, “What is the function or purpose of the fuse?”
The purpose of the fuse is to prevent overheating or fire in the event of a fault on the load side of the fuse. Electrical heating (power dissipation) is one result of electrical energy transfer. Electrical power dissipation (electrical heating) is of the form E*I, or I*I*R, or E*E/R.
To control overheating, we must have some means of controlling or limiting power dissipation. To control or limit power dissipation, we must control or limit voltage (E), current (I), or resistance (R). In most cases, the circuit is a voltage source so we cannot control the value of E.
Since we are dealing with fault conditions in the load, the value of resistance (or impedance) is obviously out of control; so, we cannot control or limit the value of R (or, more generally, impedance (Z)).
To control overheating due to electrical power dissipation, we must limit or control the value of I.
Most overheating is prevented by limiting the maximum value of I in the power equations, E*I and I*I*R.
A fuse prevents overheating by automatically reducing the value of I to zero when the current increases to a value that would cause overheating. (Current limitation must be automatic because the circuit may not be continuously attended by someone who will manually disconnect the power, and because overcurrent conditions are not necessarily immediately apparent.)
An increase in current to a value that would cause overheating is presumed to be a circuit fault-mode condition. The fuse rating or value is related to the maximum acceptable heating due to fault-mode current.
The fuse rating or value is NOT related in any way to the maximum nor-mal-mode current of a product.
Before you can select the fuse current rating, you must first have some idea of how a fuse works and what its specifications and ratings mean in terms of reducing the value of I to zero.
A fuse is comprised of a fusible link encapsulated in an enclosure and connected to contact terminals. The link is a metal which melts as a function of current and time.
As with any metal conductor, at normal temperatures the link has a low but finite value of resistance.
Current through a resistance results in power dissipation in that resistance. The power dissipation is directly proportional to the resistance and to the square of the current, as shown by the equation, P = I*I*A. The result of current in the link is power dissipation and heating of the link.
As with most metals, the link is a positive-temperature-coefficient device. That is, the resistance of the link increases with increasing temperature, and temperature increases with increasing current.
Nominally, the current rating of the fuse is the maximum current for which the link temperature is stable with time.
So, then we have our first fuse parameter, the current rating.
For currents greater than the fuse current rating, the link temperature is not stable (i. e., the temperature increases continuously), and, at some time the link will melt. Upon melting, the structure fails, the link breaks, and the current goes to zero.
Electrically, for all currents up to its rated current, the resistance of the link typically is less than 0.1 ohm. At currents greater than its rated current, the resistance of the link increases non-linearly with current and time to a very high value (hundreds of megohms), which effectively reduces the current to zero.
Whenever a circuit is opened, as the conductors separate, an arc occurs. Electrical power of the form E*I is dissipated in the arc. This power heats the ends of the broken link; the ends continue to melt back, thus creating a continuously enlarging distance between the two ends. Eventually, the distance becomes sufficiently large that the voltage cannot sustain the arc and the air becomes an insulator. (Some fuse constructions use a granular insulating material which, after the link melts, fills the space formerly occupied by the link, effectively displacing the air with a solid insulating material, thus quenching the arc.)
Now, in addition to the fuse current rating, we have three time parameters for fuse performance: melting time, arcing time, and total time (which is the sum of the melting time and the arcing time). These three times are a function of the value of the current, I. As users of fuses, melting time and arcing time can be ignored; the parameter we need is total time as a function of current Fuse manufacturers commonly publish I-t curves for each fuse current rating, where t is the total time. This is our second fuse parameter.
(When the current is very high, the magnetic field, during both melting and arcing periods, imparts energy to the highly mobile molten metal, propelling it to the wall — enclosure — of the link where it cools to its solid state. This accounts for the silver, mirror-like deposits on the inside of glass enclosures following a high-current fault.)
Note that the fuse operates by means of heating, over a period of time, of a particular conductor — the link — by electrical power dissipation in the forms I*I*R (melting) and E*I (arcing).
Electrical power dissipation over a period of time is thermal energy, and is expressed in watt -seconds, either I*I*R*t or E*I*t. We cannot expect a fuse to dissipate unlimited thermal energy; therefore, fuse ratings include a maximum current (I) rating which produces the maximum thermal energy which the fuse can safely dissipate. This maximum current rating is known by several names: interrupting rating, breaking capacity, and short-circuit rating.
Now, we have a third parameter, interrupting rating.
The interrupting rating applies to a fuse or other overcurrent device. In Europe, the terms “circuit prospective current” and “prospective short-circuit current” apply to the circuit on the supply side of the fuse. These currents are defined as the maximum current available from the supply into a short-circuit.
The fuse interrupting rating must be equal to or greater than the circuit prospective current. More about this later.
The thermal energy dissipation limit for a fuse is established by both its voltage rating and its interrupting rating in the energy equations, I*I*R*t and E*I*t.
A few years ago, I had the opportunity of participating in an experiment where we compared the performance of two fuses, both with 10,000-ampere interrupting rating, but with different voltage ratings. One was rated 250 volts, the other rated 600 volts.
We set up a short-circuit (less than 0.1 ohm) and applied 480 volts through about 5 feet of wire from a utility pole-pig transformer. (We did this test at a site belonging to the local electric utility.)
With the 600-volt fuse, the only consequence of applying the 480 volts was the open fuse.
On the other hand, with the 250-volt fuse, three inches of flame shot out of the fuseholder, the fuseholder was destroyed, board conductors were vaporized, and arcs jumped between the load-side board conductors and other unrelated board conductors. In addition, the connections from wires to the unit under test showed signs of significant arcing.
In the case of the 250-volt fuse, since the applied voltage exceeded the rating, the energy the fuse was required to dissipate was twice its rating. The fuse resistance did not increase, but, instead, let the energy through where it was ultimately dissipated by the various circuit components.
When you exceed the fuse voltage rating, the fuse does not reduce the value of I to zero. This failure of the fuse to reduce the current to zero means that electrical energy is continued to be applied to the fault, and the safety of the situation is compromised. (Note, however, that the fuse voltage rating is related to the fuse interrupting rating. The voltage rating can be exceeded, and still get acceptable fuse operation, when the circuit prospective current is very much less than the fuse interrupting rating.
One fuse manufacturer suggests the fuse voltage rating can be exceeded when the circuit prospective current is no more than ten times the current rating of the fuse. Generally, this
would be the case for most high voltage secondary circuits in electronic equipment.)
Now, we have a fourth fuse rating: the voltage rating.
In selecting a fuse, we must consider most of the fuse parameters:
1) Current rating.
2) Current-time curve ratings.
3) Interrupting current rating.
4) Voltage rating.
From the circuit, we know the circuit voltage, so we can readily choose the fuse voltage rating.
Before choosing the interrupting rating, we need to know the prospective short-circuit current of the supply circuit. Fortunately, in North America, at least, for typical supply circuits (120/240 V and 120/208Y) the prospective short -circuit currents do not exceed 10,000 amperes; all UL Listed and CSA Certified fuses — including 5 x 20 mm fuses — rated 125 V or 250 V have 10,000-ampere interrupting ratings at 125 V. So, for the most part, we need not address interrupting ratings as all North American fuses have sufficient interrupting ratings.
However, IEC 5 x 20 mm fuses do not have 10,000-ampere interrupting ratings. IEC 5 x 20 mm fuses are either 1,500- ampere or 35-ampere interrupting ratings.
(Be careful! There are TWO kinds of 5 x 20 mm fuses: some 5 x 20 mm fuses are UL-Listed to UL standards, while other 5 x 20 mm fuses are UL-Recognized to IEC standards. The UL-Listed 5 x 20 mm fuses are 10,000-ampere interrupting rating, while the UL-Recognized 5 x 20 mm fuses are either 1 ,500- or 35-ampere interrupting rating.) If supply circuits have prospective short-circuit currents up to 10,000 amperes, then what good is an IEC fuse? On the other hand, IEC fuses have been used for years with no history of detrimental operation. Why?
At the load end of an 18 AWG 2-meter power cord, the maximum current is limited by the impedance of the power cord, the plug and socket contact and other wiring contact resistances, and the building wiring impedance back to the distribution transformer, and by the size (impedance) of the distribution transformer. To get 10,000 amperes, the total impedance of the supply and return conductors must be less than 12 milliohms. The contact resistance of each wire termination is of the order of 10 milliohms, with at least 12 terminations in the circuit. Ignoring inductance, the system has 120 milliohms which limits the prospective short-circuit current to 1,000 amperes.
(I believe that the inductance is more significant and limits the current to about 100 amperes, but I don’t have the references readily available to prove it right now.)
Since the wiring of a practical installation limits the prospective short-circuit current to about 1,000 amperes, the IEC 1,500-ampere interrupting rating is adequate in most cases, and we do not see signs of detrimental fuse operation.
Finally, we come to selecting the fuse current rating and the current-time curve rating. The fuse current rating selection process is really quite simple:
First, the fuse current rating must be greater than the normal current of the load.
Second, the fuse current rating must be less than that current at which unacceptable heating occurs in the load.
The first complication is that of determining the current at which unacceptable heating occurs in the load.
The second complication is that of determining normal current as a function of time.
Let’s first look at the current at which unacceptable heating occurs in the load. Overheating results from electric energy being converted to thermal energy when current exceeds normal current. Since we are dealing with energy, and since energy includes the dimension of time, we are dealing with current exceeding normal current for some period of time. That is, we are not dealing with overcurrent situations of short duration — say less than a few seconds. Such short durations, from a practical point of view, do not usually result in sufficient energy to cause ignition. So, we are looking for a fault that results in a steady-state value of overcurrent that causes overheating.
In our search for the value of steady-state overcurrent which causes overheating, we must first identify those parts which could dissipate power and which therefore could overheat. Only those parts which can dissipate power can overheat. Parts which dissipate power include resistors, heaters, transformers, and semiconductors. Other parts, such as wire, connectors, line filters, inductors, and switches, which don’t normally dissipate power, will dissipate power under fault conditions. Both insulation and circuit component faults can cause excessive power dissipation in all of these kinds of parts. Note that the part which overheats is not the part which has the fault.
On the other hand, some parts which incur an internal fault will then dissipate power because of that fault.
Capacitors and semiconductors exhibit faults which will cause the capacitor or semiconductor to overheat. Fortunately, such faults are in the range of 0.5 to 1 ohm and therefore can be simulated with a resistor.
Once you identify candidate power-dissipating parts, you must introduce the fault which will cause the part to over-dissipate more-or-less as a steady-state condition. (This is done either with the fuse shorted out, or the highest available rating fuse in the fuse-holder.) Then, you measure the “fault-condition” input current. The fuse current rating must be less than this current.
Faults which cause unacceptable overheating are not necessarily short-circuits. In fact, short-circuits often cause some power dissipating components such as resistors and semiconductors to immediately fail open with no consequent overheating.
More often, the faults which cause unacceptable overheating are those that are of open-circuit, but finite resistance. For DC circuits, we use electronic loads as fault simulators. For AC circuits, a suitable load is a big 1:1 transformer supplied by a big variable transformer. The output of the 1:1 transformer is connected out-of phase to the AC being subjected to an overcurrent condition. Both of these schemes give a continuously variable load to the circuit under test. This process applies to both primary and secondary circuits and to both linear and switching-mode power supplies. In some cases, you will need both primary and secondary fuses.
For many switching-mode power supplies, the parts which can fault do so with relatively low impedance or resistance. For example, the forward resistance of a bridge rectifier diode is usually 1 ohm or less. Such fault impedances result in very high currents; under such conditions, the value of the fuse current rating is not critical.
However, there usually is one power resistor in the primary circuit of a switching-mode power supply. It is the resistor in the snubber circuit. Often, it is in series with a capacitor. Often the capacitor is prone to short-circuit. In such an event, the power supply continues to operate, but the power resistor must dissipate excessive power, and may cause overheating of nearby materials. The fuse current rating should be selected based on the current that occurs with the snubber capacitor shorted.
In general, for a switching-mode power supply, the fuse current rating should be selected at the next convenient current greater than the maximum input current of the switcher.
For some circuits – usually low-power circuits — the difference between normal current and fault current is less than the increment of available fuse current ratings. Transformers rated 50 watts and less, when subjected to fault conditions, often will overheat without increasing the current sufficiently to cause a fuse to protect the transformer. In such cases, a thermal switch is called for.
Now, let’s first look at the problem of determining normal current as a function of time. Most loads have turn-on currents that are higher than the steady-state current. The ideal way of selecting a fuse would be to measure the load current as a function of time from turn-on to steady-state. Using this curve, we would overlay candidate fuse curves until we found one that everywhere, from turn-on to steady-state, was just greater than the load curve.
There are two major problems with this technique. First, the conventional means of making time-dependent measurements is with an oscilloscope. The scope measures peak currents, while the fuse responds to rms currents. Second, electronic loads are usually non-linear; that is, the current is zero during a significant portion of the voltage cycle. This means that the current peaks are not in the ratio of 1.41:1 peak-to-rms as would be the case for a linear current waveform. Therefore, it is difficult to relate the measured time-current curve to the fuse time-current curve.
Since all fuses are thermal energy-operated devices, typical fuse time-current curves indicate the 10-millisecond current at 5 to 10 times the steady-state current. Typical time-delay or slow fuse time-current curves indicate the 10-millisecond current at 10 to 50 times the steady state current.
Let’s now look at “crowbar” circuits. The crowbar circuit I’m familiar with is an electronic short-circuit across the power supply output terminals. The circuit deliberately blows a fuse when the voltage of a power supply output goes too high. Its purpose is to protect IC’s from catastrophic failure from high voltage in the event of a short-circuit of the series-pass transistor in the voltage regulator circuit.
I’m a bit uncomfortable with deliberately creating an overcurrent situation — but I can’t justify my position with an engineering explanation. If the crowbar circuit is designed to carry the circuit prospective short-circuit current, then the crowbar should be okay.
Another anecdote: The original crowbar circuit worked as designed. Then, the circuit layout was changed and the SCA was relocated. As a part of the change, a connector was added to the circuit, and the wire size reduced. When the series-pass transistor shorted, the SCA fired, but the fuse did not blow. The reason: too much resistance had been added to the SCA circuit with the connector and the smaller wire. As a result, the crowbar circuit caught fire!
Some caveats: Be aware that the world has TWO small-dimension fuse rating schemes: (1) the North America (read UL and CSA) scheme, and (2) the IEC scheme. To get the same performance from either scheme, you would need to specify two different fuse rating values, one for a UL-CSA fuse, and the other for an IEC fuse. See Table 1.
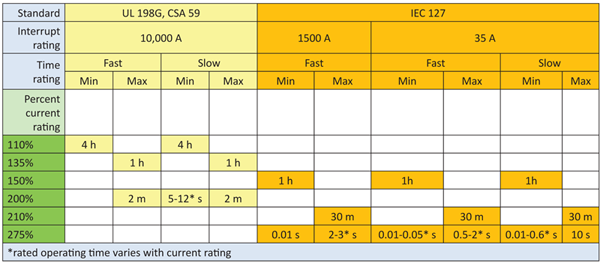
Be aware that a fuse is a temperature-dependent device; its ratings are for nominal room temperatures. A fuse should be physically located in the equipment at a point where there is little temperature rise. Or, select a larger current rating using the manufacturer’s temperature de-rating curves.
Be aware that a fuse is a temperature-dependent device; time-current curves change with physical size and, within the same physical size, with link constructions. Time-current curves are not of constant shape within fuse types. The curves will also change as a result of the heat-sinking of various kinds of fuse-holders.
Be aware that the typical “slow” fuse has a minimum time rating at 200% of its current rating, whereas the “normal” fuse has no minimum time rating at 200% of its current rating. A “slow” fuse will not blow in less than 5 seconds at 200% rating for up to 3 amperes, and will not blow in less than 12 seconds at 200% rating for fuses rated greater than 3 amperes; all other characteristics are the same as a normal fuse. See Figure 1.
Be aware that most electronic product input current waveforms are non-linear; to measure input current for the purpose of deciding a fuse value, you must measure the current with a true rms current meter.
Well… I hope this gives you some guidance for selecting a fuse value.
Mr. Nute is a Life Senior Member of the IEEE, a charter member of the Product Safety Engineering Society (PSES), and a Director of the IEEE PSES Board of Directors. He was technical program chairman of the first 5 PSES annual Symposia and has been a technical presenter at every Symposium. Mr. Nute’s goal as an IEEE PSES Director is to change the product safety environment from being standards-driven to being engineering-driven; to enable the engineering community to design and manufacture a safe product without having to use a product safety standard; to establish safety engineering as a required course within the electrical engineering curricula.