Exploring Magnetic Sensing Mechanisms in Avian Navigation
The Earth’s magnetic field is a dipole that acts like a large magnet, with its poles relatively near to the geographic (rotational) poles. Although the magnetic north pole is really in the geographic south position and vice versa, the magnetic north pole is typically referred to as the end of the dipole closest to the geographic north pole, and the magnetic south pole is similarly referred to. The geomagnetic field lines of force leave the magnetic South through Antarctica, circle the Earth, and re-enter through the magnetic North’s surface, through the Arctic pole, creating vectors of these ascending lines of force in the Southern Hemisphere and descending lines of force in the Northern Hemisphere, which are parallel to the earth’s surface at the equator.
As one moves closer to the equator, the strength of the lines of force steadily declines, reaching maximum values of around 60,000 nT at the poles and around 30,000 nT at the Equator [1]. These characteristics make the magnetic field a very reliable and omnipresent source of information, in which the magnetic vector (the vector between the line of force of the magnetic field and the line of force of gravity) provides directional information that the bird can use as a “compass.” Further, the spatial distribution of other factors, such as intensity or inclination, can be components of the “map,” providing information on the geographic position of the bird as they vary between the poles and the equator. [2, 3].
Methods of Magnetic Sensing in Birds
Although it is not completely understood how migratory birds are capable of sensing the orientation and intensity of the geomagnetic vector field generated by the Earth, behavioral experiments indicate that they use a combination of sensing methods and that the combination of these senses provides migratory birds with the ability to successfully migrate [4]. Referred to as the avian magnetic compass, migratory birds determine their position and direction with two separate measurements, which consist of inclination (or deviation) from the magnetic field lines for determining orientation with the poles and magnetic field intensity for determining direction [4]. The two primary methods of detecting inclination and intensity are the radical pair mechanism and the magnetite hypothesis.
Radical Pair Mechanism
A likely mechanism for an axial magnetic compass in migratory birds, the radical pair mechanism relies on unpaired electrons with parallel (T) and antiparallel (S) spins [5]. Sets of unpaired electrons result in differing chemical properties based on what spin combination the set has. This, in turn, alters the reaction rate and yield of chemical processes that occur. A weak external magnetic field can modify the relative alignment of the electrons, thereby altering the reaction rate and yield, and is dependent on the intensity and the orientation of the external field.
For migratory birds, this process usually occurs on the surface of certain cell membranes. The direction of the external field lines produces arrays of protein oriented in the same direction, and the varying density of the synthesized protein enables the bird to determine the orientation of the field lines [5].
In order to produce these radicals, cryptochrome photoreceptors located in the eyes of the bird use photons from external light, such as the sun [4]. Behavior experiments determined that the photoreceptors and the bird’s ability to navigate are impacted by the wavelength of perceived light. Further, short wavelengths of light from UV to about 560nm were necessary for radial pair production [4]. Tests that involved birds in total darkness displayed a 90-degree shift in the preferred direction, which suggested that the radical pair sensing mechanism was not activated. Instead, a separate magnetic sensing mechanism behaves as a backup [5]. Although it is uncertain what the backup mechanism is, it likely relies on the magnetite-based magnetoreception mechanism.
Magnetite Hypothesis
The biomineralization of magnetite in animals that migrate, such as certain birds and fish, leads to the hypothesis that the ferrous ferrite may play a role in magnetoreception [5]. Crystals of magnetite roughly 50nm in size are attached to mechanoreceptors within specific cells and behave as small compass needles within the cells of the bird or fish. The torque produced by the crystal under an external magnetic field triggers the receptor, thereby providing a method of sensing the field. This behavior has also been studied in magnetotactic bacteria, which orient themselves with external magnetic fields [5].
Studies conducted on homing pigeons have determined that magnetite-containing dendrites are located at six locations on the upper beak. Clusters of the dendrites have been found to deform under weak magnetic fields, producing a torsion on the dendrite. We hypothesize that this torque behaves in the same manner as the radical pairs, and provides a complementary sensing method for magnetic fields [5].
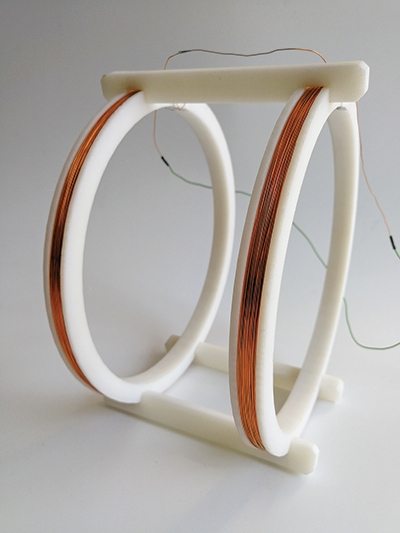
Magnetic Pulse Generation with Helmholtz Coil
In order to further investigate the behavior and functionality of magnetoreception in birds (or other animals), the application of magnetic pulses with specific direction and intensity are used to study how the bird’s flight behavior is affected. The application of magnetic shielding techniques may be used to block Earth’s existing magnetic field. But in order to generate a uniform magnetic field with specified direction and intensity, the application of a Helmholtz coil is employed [6].
Two coils placed in parallel along the same axis can produce a relatively uniform magnetic field between the coils, This configuration is known as a Helmholtz coil, named after the German physicist Hermann von Helmholtz. Three pairs of Helmholtz coils can be configured on the X, Y, and Z axis, allowing for complete control of the magnetic field in the center of the configuration.
A configuration of a 3D Helmholtz coil system can be seen in [6]. This configuration enables the ability to cancel Earth’s magnetic field, as well as produce a static, rotating, or alternating field [6].
Effects of Magnetic Pulses on Birds
The application of 500mT pulses for a few seconds on passerine migrants (bird) initially produces a shift in orientation during flight [5]. As expected, by 4 to 10 days after the treatment, the passerine migrants that had experienced the pulses had recovered their normal orientation and returned to their expected migration path. Another important note made in the experiment was that young birds that had not yet migrated were not affected by the pulse treatment. The shift in orientation due to magnetic pulses indicates that there is involvement with magnetic material for migratory and targeted-location flight [5].
A similar experiment was conducted on homing pigeons [5]. It was determined that pigeons treated with pulses deviated from the untreated control group path, a deviation that became more substantial as the distance between home and the release point was increased. Overall, this indicated that the magnetic pulses would affect the internal avian compass but left the navigation map relatively unaffected, as the pigeons were still capable of returning home.
Conclusion
The magnetic orienting mechanism in birds has been discovered to be a two-step system. First, utilizing the information offered by variables of the terrestrial magnetic field, such as strength or tilt, the map allows them to calculate their geographical position, and the compass allows them to decide the direction to follow. It is also known that birds have an intrinsic sense of magnetoreception. It is the primary foundation, along with the internal circadian clock, for establishing the many navigation systems through intricate learning processes. All of this comes together to produce an adult bird’s entire navigation system.
The following are two hypothesized magnetoreception models: By converting the strength of the Earth’s magnetic field into mechanical force within specialized cells, magnetoreception based on magnetite particles housed in the upper part of the beak function as chains of magnetite particles that interact with the Earth’s magnetic field and provide directional information and even geographic position to the birds.
Chemical magnetoreception based on a radical pair model, in which a molecule is energized by the absorption of a photon, produces an electron and forms a pair of radicals, which affects the speed of singlet-triplet interconversion depending on the alignment with the Earth’s magnetic field.
Because both magnetoreception models are hypothetical, the current understanding of magnetoreception models is insufficient to identify the magnetoreception model employed. Although a clear picture of how information from the magnetic compass is interpreted is beginning to appear, our present understanding of magnetoreception is constantly evolving.
There are still a lot of questions concerning magnetoreception and how data is processed from these receptors to the brain. Advances in behavior, anatomy, and physiology will aid in the discovery and identification of magnetic reception structures in the future.
References
- W. H. Campbell, Introduction to Geomagnetic Fields, 2003.
- R. Wiltschko and W. Wiltschko, “Avian navigation: From historical to modern concepts,” Animal Behaviour, vol. 65, no. 2, pp. 257–272, 2003.
- R. C. Beason, “Mechanisms of magnetic orientation in birds,” Integrative and Comparative Biology, vol. 45, no. 3, pp. 565–573, 2005.
- R. Wiltschko and W. Wiltschko, “Magnetoreception in birds,” Journal of The Royal Society Interface, vol. 16, no. 158, p. 20190295, 2019.
- M. Winklhofer, “The physics of geomagnetic-field transduction in Animals,” IEEE Transactions on Magnetics, vol. 45, no. 12, pp. 5259– 5265, 2009.
- N. Aldoumani, T. Kutrowski, J. Barnes, T. Meydan, and J. T. Erichsen, “Instrumentation to investigate the magnetoreception of homing pigeons by using applied magnetic fields,” IEEE SENSORS 2014 Proceedings, 2014.