A Dozen Tips for Successful Designs
The automotive industry and individual automobile manufacturers must meet a variety of electromagnetic compatibility (EMC) requirements. For example, two requirements state that electronic systems must not emit excessive electromagnetic interference (EMI) or noise, and must be immune to noise emitted by other systems. This article explores some of these requirements and offers some tips and techniques that you can use to help ensure that designs for equipment such as infotainment or advanced driver assistance systems are compliant with these requirements.
Overview of EMC Requirements
Comité International Spécial des Perturbations Radioélectriques (CISPR) 25 is a standard that presents several test methods, with suggested limits, to evaluate the level of radiated emissions from a component designed for installation in a vehicle.1,2 In addition to the guidance that CISPR 25 provides to manufacturers, most manufacturers have their own set of standards to augment CISPR 25 guidelines. The primary purpose of CISPR 25 testing is to ensure that a component will not interfere with other systems within the vehicle.
CISPR 25 requires that the electromagnetic noise level in rooms where its tests are performed must be at least 6dB lower than the lowest levels being measured. Since CISPR 25 has test methods where it looks for levels as low as 18dB (microvolts per meter), that requires an ambient level of less than 12dB (microvolts per meter). As reference, 12dB is approximately the field strength of a typical AM radio station located 1km from the antenna.3
In today’s environment, the only way to meet this requirement is to perform testing in a special chamber designed and built to shield the testing environment from outside fields. Due to the small room size, it is also important to protect this environment from reflections of signals generated within the room. The test chamber walls must be lined with a material that will not reflect electromagnetic waves (see Figure 1). Test chambers are expensive and typically rented by the hour. To save costs, it is a good idea to evaluate EMC and EMI issues during the design phase to achieve first-time success in the chamber.
Another testing standard is the International Organization for Standardization (ISO) 11452-4 bulk current injection (BCI) suite of tests, which verify whether a component is adversely affected by narrowband electromagnetic fields. Testing entails inducing disturbance signals directly into wiring harnesses with a current probe.
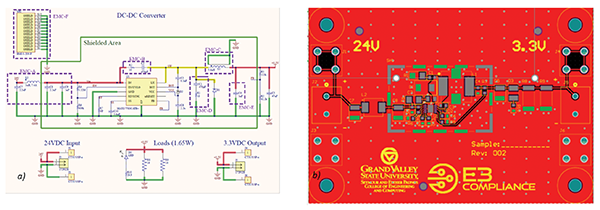
12 Tips for Successful EMC Testing
#1 Keep loops small
When a magnetic field is present, a loop of conductive material acts as an antenna and converts the magnetic field into a current flowing around the loop. The strength of the current is proportional to the area of the enclosed loop. Therefore, as much as possible, keep loops from existing, and keep any required enclosed areas as small as possible. An example of a loop that might exist is when there is a differential data signal. A loop can form between the transmitter and the receiver with the differential lines.
Another common loop is when two subsystems share a circuit, perhaps a display and an engine control unit (ECU) driving the display. There is a common ground (GND) connection in the chassis of the vehicle – a connection to this GND at the display end and at the ECU end of the system. When the video signal connects to the display with its own ground wire, it can create one huge loop within the ground plane. In some cases, a loop like this is unavoidable. However, by introducing an inductor or a ferrite bead in the connection to GND, a DC loop can still exist; but from a radio-frequency (RF) emissions standpoint, the loop is broken.
Also, every differential driver/receiver pair forms a loop when a signal travels over a twisted-pair cable. Generally, this loop has a small area for the cable portion of the link because the twisted pair is tightly coupled. However, once the signal gets to the board, you should maintain close coupling to avoid opening up the loop area.
#2 Think About the Frequencies Used in the System
Often there will be multiple links carrying similar information – for example, a camera system may have a camera carrying video from the back of the vehicle to a processor, and then there will be a similar link carrying the video to a display. If both signals are running at the same frequency and phase, there is an opportunity for them to constructively interfere, and generate larger interference spurs than either could produce on its own. One way to handle this might be to use slightly different frequencies in the two links, or different phases when transporting similar signals.
#3 Select Frequencies That Will Not Interfere with Sensitive Systems
Some systems in an automobile are more sensitive than others. CISPR limits have specific bands to prevent interference from things like FM radio broadcasts and GPS. Since GPS signals are often very weak, the GPS limits tend to be much tighter. Avoiding clock frequencies that are exact submultiples of these sensitive bands will help place interference spurs generated in-between the most sensitive frequency bands, rather than on top of them.
#4 Bypass Capacitors are Essential
Complementary metal-oxide semiconductors (CMOS) circuits are very popular, in part because of their high speed and very low power dissipation. An ideal CMOS circuit only dissipates power when it is changing states and when the node capacitances need to charge or discharge. From a power-supply standpoint, a CMOS circuit that requires 10mA on average may be drawing many times that during clock transitions, then little or no current between cycles. Therefore, emission-limiting techniques are focused on peak voltage and current values rather than average voltage and current values.
Current surging from the power supply to the power pin on a chip during clock transition is a prime source for emissions. By placing a bypass capacitor close to each power pin, the current required to supply the chip during the clock edge comes directly from the capacitor. Then the charge on the capacitor builds up with a lower, steadier current between cycles. Larger capacitors are good for supplying large surges of current, but tend to react poorly to very high-speed demands. Very small capacitors can react quickly to demand, but their total charge capacity is limited and can quickly become exhausted. The best solution for most circuits is to use a mix of different-sized capacitors in parallel, perhaps 1µF and 0.01µF capacitors in parallel. Place smaller-size capacitors very close to the chip’s power pins, and larger-size capacitors further away.
#5 Good Impedance Matching Minimizes EMI
When a high-speed signal is sent through a transmission line and encounters a change in the characteristic impedance on that line, part of the signal is reflected back to the source of the signal and part continues along in the original direction. Invariably, the reflection leads to emissions. For low EMI, good high-speed design practice is a necessity. There are a plethora of good sources for transmission-line design information.4,5 Here are some suggested precautions when designing transmission lines:
Remember that the signal exists between the ground plane and the signal trace. Either an interruption in the signal trace or the ground plane can cause emissions, so pay attention to ground-plane cutouts or discontinuities beneath the signal trace.
Try to avoid sharp angles on the signal trace. Nicely curved corners are much better than right-angle turns.
Often, an FPD-Link signal will have components tapped off of it, such as power over coaxial cable, power connections, AC-coupling capacitors and many others. To minimize the reflections at the components, try to use small components such as the 0402 size and set the width of the trace to be the same as the width of the 0402 component pad. Also, be sure to set the characteristic impedance of the trace by controlling the dielectric thickness in the stackup.
#6 Shielding
Do not shortcut good shielding techniques. When designing to minimize emissions, put a shield around the offending portion of the circuit. It may still
emit energy, but good shielding can capture the emissions and send them to GND before they escape from the system. Figure 2 illustrates how shielding can control EMI.
Shielding can take a variety of forms. It might be as simple as enclosing a system in a conductive case, or it could involve fashioning small custom metal enclosures that are soldered over emission sources.
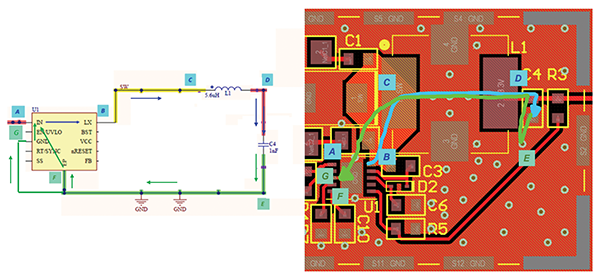
#7 Short Ground Connections
Every bit of current that flows into a chip flows back out again. Several tips in this article discuss having short connections to the chip – bypass capacitors close to the integrated circuit (IC), keeping loops small, etc. However, often forgotten is the path that the ground current has to take to get back to its source. In an ideal situation, you can dedicate a layer of the board to GND so the path to GND is not much longer than a via. However, some board layouts have cutouts in ground planes that can force ground currents to take a long path from the chip back to the power source. While the GND current is taking this path, it is acting as an antenna to transmit or receive noise.
#8 No Faster Than Needed
There is a tendency to worry about timing margins and to use the fastest logic possible to provide the best timing margins. Unfortunately, very fast logic has sharp edges with very high-frequency content that tends to produce EMI. One way to reduce the amount of system EMI is to use the slowest logic possible that will still meet timing requirements. Many field-programmable gate arrays (FPGAs) allow you to program the drive strength at lower levels, which is one way to slow the edge rates. In some cases, series resistors on logic lines can decrease the slew rates of signals in the system.
#9 Supply-Line Inductors
Tip #2 discussed bypass capacitors as a way to decrease the impact of current surges. Inductors on the supply lines are another side of the same coin. By placing an inductor or ferrite bead on a power-supply line, it forces the circuits connected to that supply to draw their dynamic power requirements from the bypass capacitors, rather than all the way back from the power source.
#10 Caps at Inputs to Switching Supplies
One recurring theme when looking to solve EMI issues is to reduce dv/dt and/or di/dt wherever possible. In this context, DC/DC converters may seem completely harmless until you realize that they don’t convert directly from DC to DC. Rather, they go from DC to AC to DC. Hence, the AC in the middle has the potential to cause EMI problems.
One area where automotive designers are concerned about creating interference is in the AM radio band. Almost every automobile is equipped with an AM radio, which has a very sensitive high-gain amplifier tunable from 500kHz to 1.5MHz. If a component is emitting a signal within this band, it will probably be audible on the AM radio. Many switching power supplies use switching frequencies within this same band, which leads to issues in automotive applications. As a result, most automotive-switching supplies use switching frequencies that are above this band – often at 2 MHz or higher. If there is insufficient filtering either at the input or the output of a switching power supply, some of this switching noise may find its way into other subsystems that may be sensitive to the root or subharmonic frequencies.
#11 Watch for Resonances
Various inductors and capacitors can tame the dv/dt and di/dt evils that can lead to EMI. However, inductors and/or capacitors can have undesirable characteristics related to self-resonance. You can often rectify this problem by adding a resistor in parallel to the inductor to absorb the energy of the oscillation before it becomes big enough to cause issues.
Another potential issue is when there is a series inductor – either a discrete component or a parasitic inductance from a power line – that leads to a component with a bypass capacitor. The resulting inductor-capacitor (L-C) circuit has the potential to oscillate at the resonant frequency. Once again, you can alleviate this with a resistor, often placed in parallel with the inductor.
#12 Spread-Spectrum Clocking Reduces Peak Emissions
With components, such as FPD-Link serializers and deserializers (SerDes), there is often a data bus and clock that have the option of spread-spectrum clocking. In spread-spectrum clocking, the clock signal is modulated. The result is that energy generated by the edges of the clock and data signals spreads across a wider frequency band than it would otherwise occupy. Since EMI specifications are set to limit peak emissions at any frequency within a band, spreading noise across a wider band can help minimize the noise peaks.
A good example of a deserializer is the DS90UB934-Q1, which is often used in conjunction with the DS90UB913A-Q1 serializer. These devices provide a video link between a camera in an advanced driver assistance system (ADAS) and the processor. The deserializer recovers the clock that the image sensor in the camera provided to the serializer and outputs this clock along with the data for use by the processor. Ten or 12 high-speed data lines that transition concurrently with a high-speed clock are a prime source of EMI. To mitigate this EMI, the DS90UB934 has an option to use a spread-spectrum clock with the output data, rather than the lower-jitter clock that the image sensor provides. The spread-spectrum clock is controlled through registers in the deserializer.
Conclusion
As automobiles rely more on electronics for critical vehicle operation in addition to entertainment and comfort functions, there is a growing need to operate without error in the presence of interference and to not provide interference to other systems within the vehicle. By following the tips and techniques outlined in this article, and by selecting appropriate components, you can design robust systems that will enable the reliable operation of automotive systems without EMI problems.
Endnotes
- CISPR 25 Ed. 4.0 b:2016: Vehicles, boats and internal combustion engines – radio disturbance characteristics – Limits and methods of measurement for the protection of on-board receivers.
- Rodriguez, Vincente. “Automotive Component EMC Testing: CISPR 25, ISO 11452-2 and Equivalent Standards.” Safety & EMC 2011, 26-34.
- Federal Communications Commission AM Groundwave Field Strength Graphs – Sections 73.183 and 73.184.
- Wadell, Brian C. “Transmission Line Design Handbook.” Boston: Artech House, 1991.
- Johnson, Howard W., and Martin Graham. “High-Speed Signal Propagation: Advanced Black Magic.” Upper Saddle River, New Jersey: Prentice Hall Professional, 2003.
Mark Sauerwald is an applications engineer with Texas Instruments and has worked in the semiconductor component industry for 30 years. He holds a degree in electrical engineering from the University of California at San Diego, and can be reached at mark.sauerwald@ti.com.