Many of my clients are power electronics companies that specialize in making switched-mode power supplies and motor drives. During the conducted emission tests, one of the challenges these manufacturers face is the resonance peaks in the harmonic noise somewhere between 10 MHz and 30 MHz. Often, no amount of filtering will eradicate or attenuate the peak. This is so typical that it is worth investigating the root causes of these peaks.
What Caused the “Hump”?
In Reference 1, Williams explains in great detail that the “hump” in the 20 MHz is caused by the structural resonance introduced by the test set-up. The circuit under test normally has a front-end filter which often consists of common-mode capacitors and inductance. The main power conversion stage usually utilizes magnetic components such as a transformer or inductor, which itself has parasitic capacitance. The DUT is connected to a LISN by a cable (often longer than 1 meter), so the common mode transfer function of the set-up (including the circuit, the cable, and the LISN) has a few poles and zeros, indicating multiple resonance points.
When the circuit is energized with multiple energy sources (hard-switching devices in the circuit), it will show the resonance peak in the test results. As in the 10s of MHz frequency range, it is the rise time of the switching event that determines the energy level. It is safe to conclude that the resonance peak is caused by how hard the switching event is (rise time) rather than how often it is switched (frequency).
It is also worth mentioning that in 10s of MHz, the conducted emission of a switched-mode power supply is predominantly common mode.
How to Locate the Noise Source
As explained earlier, a switched-mode power supply often consists of multi-stage converters, which means there could be multiple noise sources. A typical example is an AC-DC converter, where the AC to DC high-voltage (HV) stage often includes a power factor correction (PFC) circuit (buck or boost type). From the DC HV to DC low-voltage (LV) stage, the circuit can be a flyback, an LLC, a dual active bridge (DAB) or a phase-shift full bridge, depending on the power level. In this particular case, design engineers often face challenges in determining whether the resonance peak is caused by the PFC circuit, the DC-DC converter, or both.
To locate the noise source, it is essential to understand the relationship between the time domain and the frequency domain. A resonance peak observed in the frequency domain can also be observed in the time domain. Wyatt provides a demonstration of this concept in a video [2].
Here, we present a case study demonstrating how to locate the noise source in a motor drive circuit. The method is based on techniques introduced in Reference [3]. In Figure 1, we can observe a 10 MHz resonance peak in the conducted emission results. The motor drive unit comprises an AC-to-DC power converter stage (with active power factor correction), a DC-to-DC power converter stage, and a DC-to-three-phase motor drive circuit. It is crucial to identify which circuit in this product is responsible for the 10 MHz “hump.”
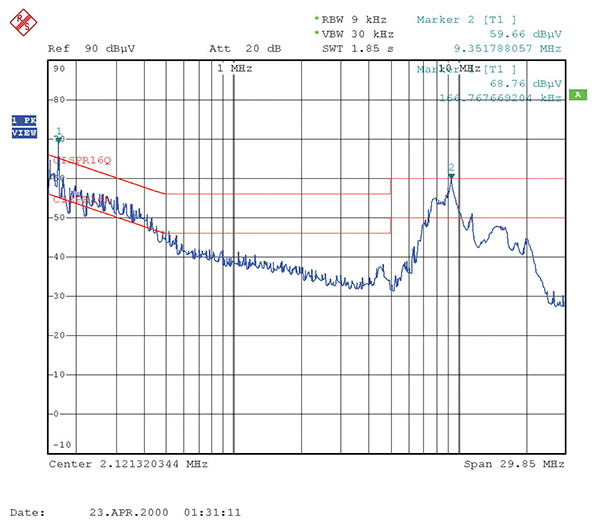
Figure 2 illustrates the setup for locating the noise source. An RF current probe is positioned on the mains cable and connected to the 50-ohm input of an oscilloscope (channel 2 in this case). Channel 1 of the oscilloscope (also configured to be a 50-ohm input) is connected to a near-field probe. The measurement result from the RF current probe serves as a stable trigger. By moving the near-field probe to accessible parts of the system, we can compare the noise signal picked up by the near-field probe with the RF current probe signal. The noise signal picked up by the near-field probe can be in phase or anti-phase compared with the RF probe.
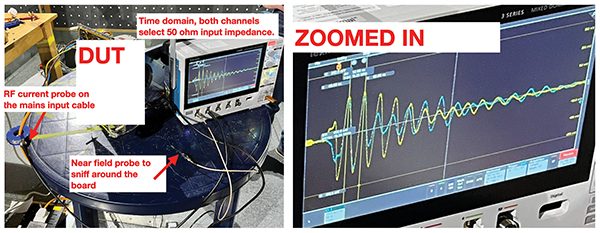
After zooming in on the noise signal captured by the RF current probe, we can observe the 10 MHz ringing on the oscilloscope. If the near-field probe also displays a similar ringing pattern, we can conclude that the noise source has been located. In Figure 2, it is evident that both channels exhibit a 10 MHz ringing when the near-field probe is positioned near the PFC circuit.
Once the noise source is identified, we can proceed to develop strategies for mitigating its impact. In this case, the solution involves optimizing the RC snubber circuit of the PFC circuit, which utilizes a SiC MOSFET. The high noise level experienced can be attributed to the SiC MOSFET. By implementing improvements to the RC snubber circuit, we can effectively reduce the noise generated by the PFC circuit.
References
- Williams, EMC for Product Designers, Fifth edition, Newnes, 2017.
- Wyatt, “Switch-Mode Power Supply – Ringing Demo.”
- C. Smith, High Frequency Measurements and Noise in Electric Circuits, Springer, 1992.