This article describes a laboratory experiment that shows the impact of the decoupling capacitors and a PCB trace length on the signal integrity in a CMOS inverter circuit.
Switching Circuit Description
Figure 1 shows the block diagram of the switching circuitry.
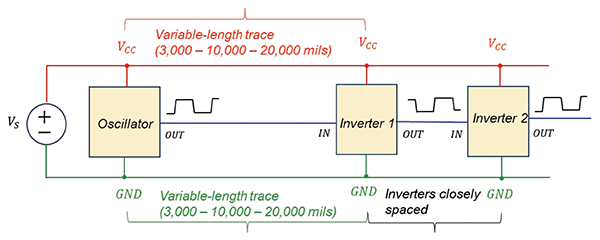
A detailed schematic of the circuit is shown in Figure 2.
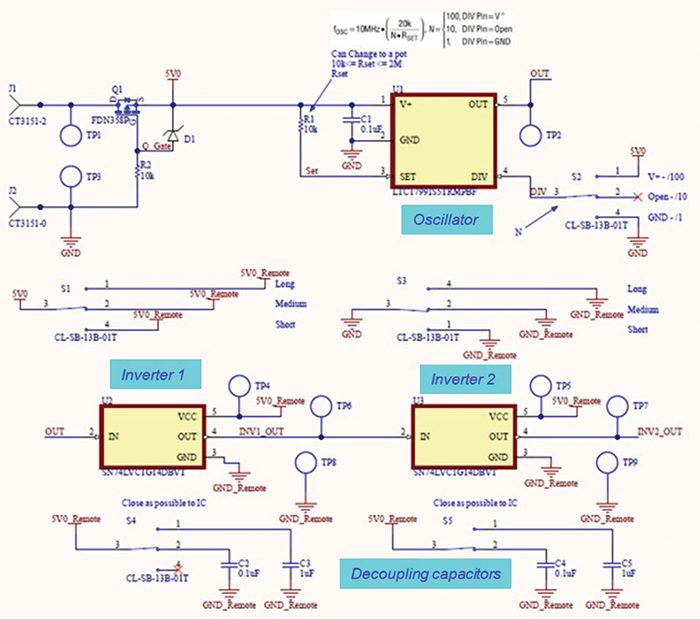
The PCB used in this experiment is shown in Figure 3.
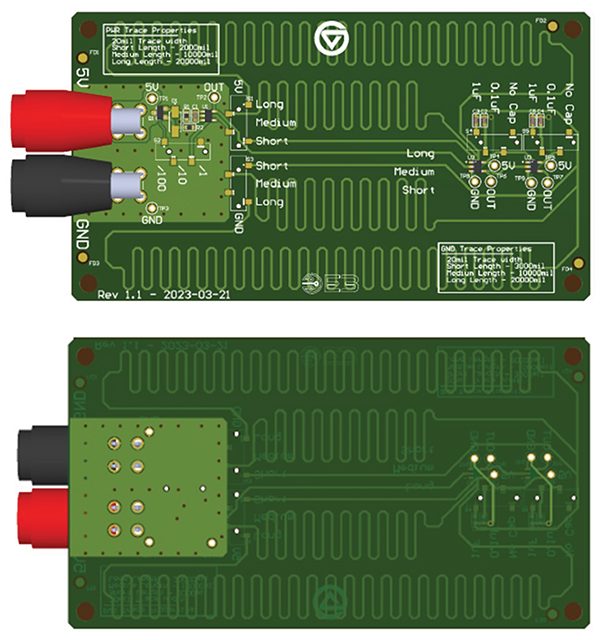
The PCB used in this experiment is a newer version of the board used previously and described in [1]. This new PCB (designed by Mathew Yerian-French) contains several switches allowing the user to change the oscillator frequency, the length of power, and ground traces and choose whether to use the decoupling capacitors.
During switching, a transient current is drawn from the source. The inductance associated with the current loop causes the voltages at the power and ground pins to deviate from the desired values, as shown in the measurement section (see [1] for more details).
The board was purposely designed with very long traces to show the negative impact of the associated inductance while increasing the impact of a decoupling capacitor at the same time.
Impact of the Trace Length and Decoupling Capacitors: Measurements
The measurement setup is shown in Figure 4 (see [2] for the experiment details, Altium board files, and BOM).
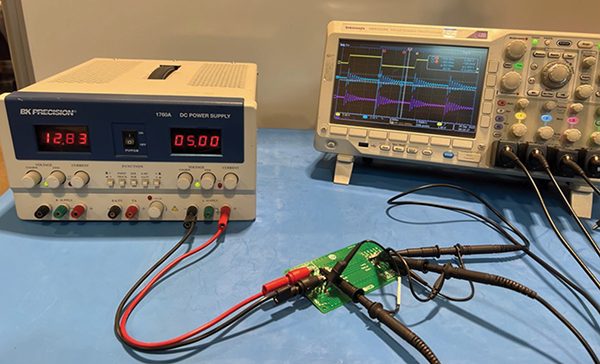
Figures 5 and 6 show the voltages at the VCC pins of the inverters with respect to their GND pins. Figure 5 shows the results for short traces, while Figure 6 shows the results for long traces with no decoupling capacitors.
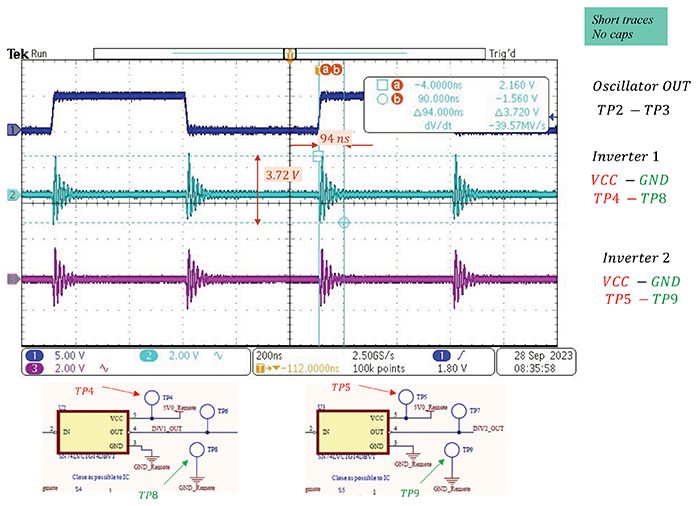
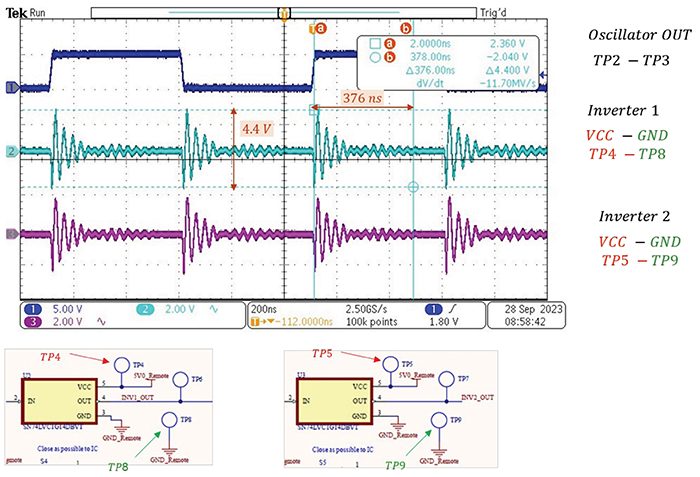
Observations: Both the amplitudes and duration of the ringing are larger for long traces as compared to short traces.
Figures 7 and 8 show the voltages at the VCC pins of the inverters with respect to their GND pins for short traces vs. long traces with a 0.1 µF decoupling capacitor at each inverter.
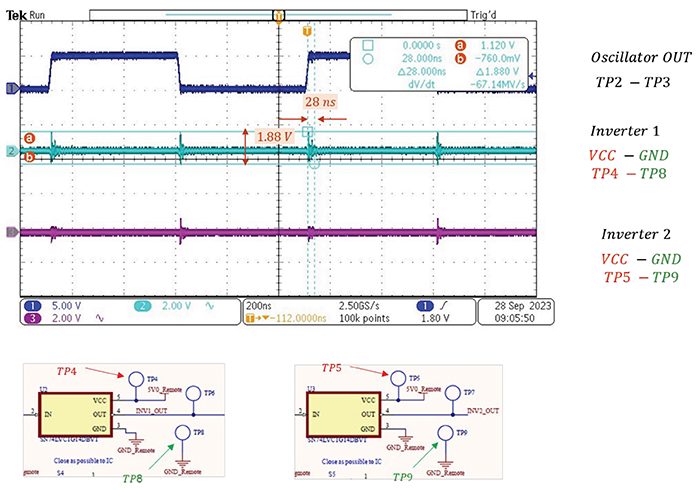
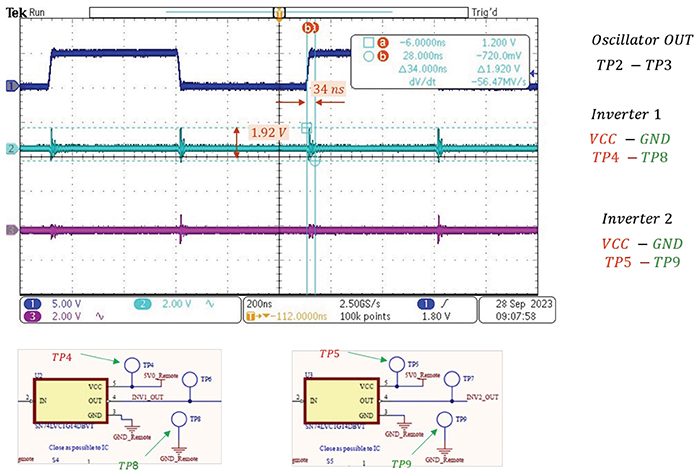
Observations: Decoupling capacitors dramatically reduce the ringing amplitudes and duration. The impact of the trace length is not as pronounced.
Figures 9 and 10 show the voltages at the GND pins of the inverters with respect to the main circuit input GND for short traces vs. long traces with no decoupling capacitors.
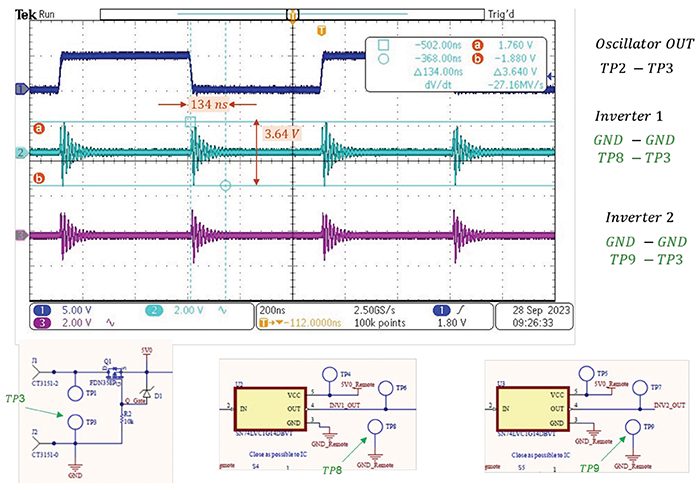
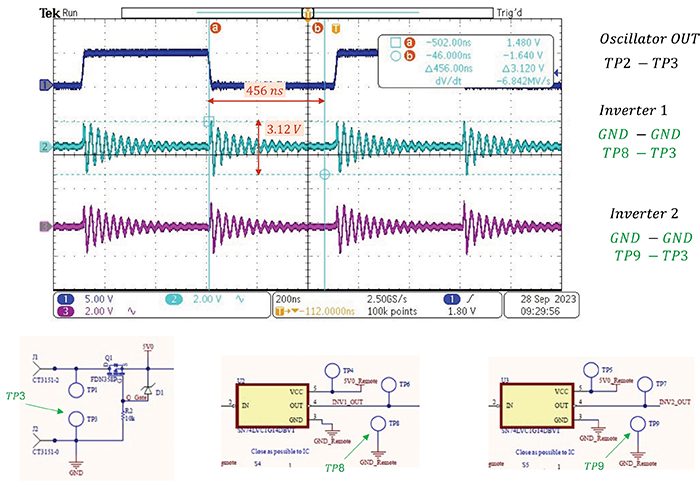
Observations: The duration of the ringing for long traces is significantly larger as compared to short traces. The amplitude of the ringing for long traces is, however, not as large as for short traces.
Figures 11 and 12 show the voltages at the GND pins of the inverters with respect to the main circuit input GND for short traces vs. long traces with a 0.1 µF decoupling capacitor at each inverter.
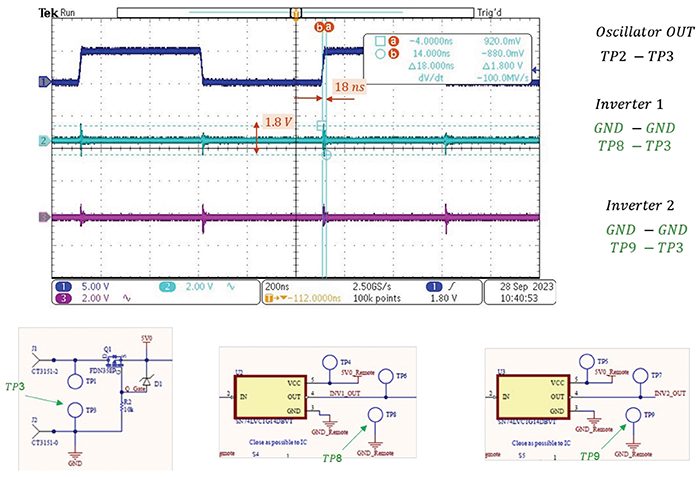
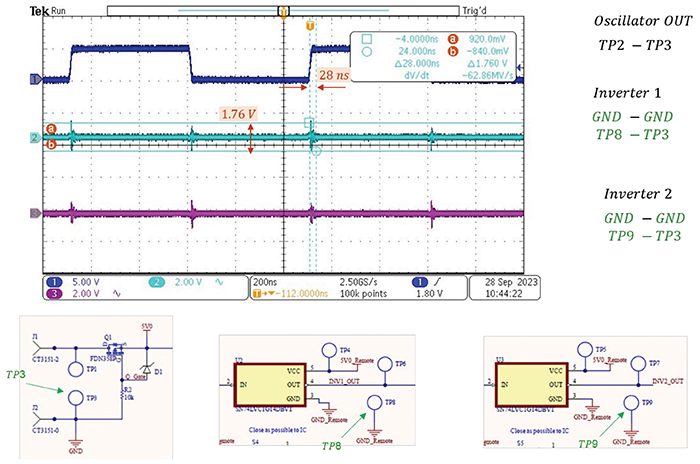
Observations: Decoupling capacitors dramatically reduce the ringing amplitudes and duration. The impact of the trace length is barely noticeable.
All measurement results are summarized in Table 1.
VCC – GND ∆V [V] |
VCC – GND ∆t [ns] |
GND – GND ∆V [V] |
GND – GND ∆t [ns] |
|
Short trace, no caps | 3.72 | 94 | 3.64 | 134 |
Long trace, no caps | 4.4 | 376 | 3.12 | 456 |
Short trace, 0.1µF caps | 1.88 | 28 | 1.8 | 18 |
Long trace, 0.1µF caps | 1.92 | 34 | 1.76 | 28 |
Table 1: Summary of the results
Future Work
The next column in the February issue describes the operation of an ideal difference amplifier.
The upcoming March 2024 column will discuss the impact of a decoupling capacitor and trace length on radiated emissions. Measurements will be performed in a semi-anechoic chamber, in accordance with CISPR 25 Edition 5 automotive standard, using a monopole antenna was used in the frequency range of 150 kHz – 30 MHz, a biconical antenna in the range of 30 MHz – 300 MHz, and a log-periodic antenna in the range of 300 MHz – 1GH.
The April 2024 column will be devoted to conducted emissions measurements in accordance with the same standard. Measurements will be performed in the same semi-anechoic chamber, both on the battery and ground lines in the frequency range of 150kHz – 108 MHz.
References
- Bogdan Adamczyk, “Impact of a Decoupling Capacitor in a CMOS Inverter Circuit,” In Compliance Magazine, September 2019.
- Bogdan Adamczyk, Principles of Electromagnetic Compatibility: Laboratory Exercises and Lectures, Wiley, 2023.