Conducted RF emissions are electromagnetic disturbances (noise voltages and currents) caused by the electrical and electronic activity in an item of equipment, and conducted out of that equipment along its interconnecting cables, such as power, signal or data cables. Sometimes the conducted emissions are due to the activity of circuits connected directly to a particular conductor, and sometimes they are coupled into that conductor from unrelated circuits inside the equipment, due to common-impedances, electric or magnetic fields, or even electromagnetic fields.
Author’s Note: Since this guide was first written, the standard it covers has been updated and some details may have changed. Always use the relevant version of the test standard.
What are conducted RF emissions and how are they caused?
Conducted RF emissions are electromagnetic disturbances (noise voltages and currents) caused by the electrical and electronic activity in an item of equipment, and conducted out of that equipment along its interconnecting cables, such as power, signal or data cables. Sometimes the conducted emissions are due to the activity of circuits connected directly to a particular conductor, and sometimes they are coupled into that conductor from unrelated circuits inside the equipment, due to common-impedances, electric or magnetic fields, or even electromagnetic fields.
The conducted disturbances in a particular conductor, emitted by one item of equipment, can couple directly into another item of equipment that is connected to the same conductor. Conducted disturbances are also radiated from the conductors they travel along, as both electric and magnetic waves, and in this sense, the conductor is acting as an ‘accidental transmitting antenna’.
Fluctuating voltages in a conductor of any type creates conducted electric waves in the air and other insulators (dielectrics, e.g. plastic cable insulation). Fluctuating currents in a conductor of any type creates magnetic waves. After travelling for a distance equivalent to approximately one-sixth of their wavelength, both electric and magnetic waves have turned into electromagnetic (EM) waves, comprising electric and magnetic waves travelling together with intensities in a ratio known as the ‘wave impedance’; appropriate to the medium they are travelling in. These electromagnetic waves are sometimes also called plane waves.
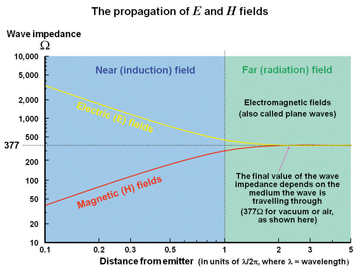
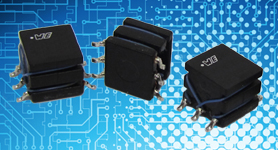
The strength of the waves as they spread out in space varies, creating an electromagnetic field. Electric field strengths are measured in Volts/metre (usually dBµV/m for emissions measurements, where 0dBµV/m = 1µV/m). Magnetic field strengths are measured in Amps/metre (usually dBµA/m for emissions measurements, where 0dBµA/m = 1µA/m). The creation of magnetic waves from electric waves (and vice-versa) is a bit like Ohms law – pass a current through a resistor and a voltage will appear – apply a voltage across a resistor and a current appears. In fact, the formula: ZW = E/H (where ZW is the wave impedance of the medium, E is the electric field strength in Volts/metre and H is the magnetic field strength in Amps/metre) is often described as “Ohms Law for fields”. The ZW for air (and vacuum) is almost exactly 377Ω.
If we measure our field strengths in the ‘far field’, at distances greater than one-sixth of a wavelength (no closer than 1.5 metres at 30 MHz), we can choose whether to measure E or H, since they have a fixed relationship with each other (ZW). Typically we measure the electric field (dBµV/m), when we are measuring conducted emissions at frequencies above 30 MHz. However, when we need to know the E and H fields at distances closer than one-sixth of a wavelength (known either as the ‘near field’ or ‘induction field’) we need to use both electric and magnetic field transducers because it is hard to predict the complex relationship between the two quantities in this region.
These waves pass through the air, vacuum, and insulating materials such as wood, brick, plain plasterboard, concrete, plastic, fiberglass, etc. Every type of electronic equipment ‘leaks’ such fields, either unintentionally, or because it is an intentional radio transmitter.
Radio, TV and radar transmitters, and industrial scientific or medical equipment (ISM) that uses RF energy to perform their direct functions (covered by CISPR 11 or EN 55011), can emit very powerful fields, at the radio frequencies they are permitted to employ. But the focus of emissions testing using EN 55022 is on unintentional emissions, not intentional transmitters. EN 55011 sets limits for the emissions from ISM equipment, at the specified ISM frequencies as well as at the other frequencies in its range.
CISPR 22, EN 55022, CISPR 11 and EN 55011 measure the emissions from equipment in the frequency range 150 kHz to 30 MHz simply by using a conducted measurement technique on the AC mains supply cable. The conducted emissions limits applied by these standards are intended to protect equipment connected to the same AC mains supply, and also are assumed to protect the radio communications spectrum below 30 MHz from the fields radiated by an equipment’s AC supply cables. These standards do not test the conducted emissions from other cables, so they obviously cannot completely define all of an equipment’s possible effects on its electromagnetic environment.
As frequencies fall below 30 MHz any reasonable antenna distance soon becomes in the near field, so it would become necessary to make two measurements – one of the E field and one of the H field – to completely define the emissions in this frequency range. Some military standards employ such near-field radiated field measurements as well as conducted emissions tests on AC mains supply and other cables.
What problems are caused by conducted RF emissions?
Noise voltages and currents can, of course, be conducted directly from an item of interconnected equipment, via the AC mains supply cables they share, or via any other conductors such as signal or data cables. But conducted emissions are also radiated into the environment, and the resulting RF fields can affect equipment that does not share any conductors with the source of the noise. When wires, cables, connectors, and any other conductors are exposed to E, H or EM fields – currents and voltages are induced in them – the conductor behaves as an ‘accidental receiving antenna.’
All of the conducted and accidentally received currents and voltages are noise as far as the victim equipment is concerned, with the potential ability (if at too high a level and/or at certain frequencies) to cause unacceptable variations in its circuits’ electrical and electronic performances (including misoperation of software). When the variations caused by the induced electrical noise are too great, we say that the equipment is suffering from electromagnetic interference (EMI) or, sometimes, just interference.
Obviously, when the noise voltage or current is within the operating frequency range of a circuit, a sufficient level of noise will affect the circuit. But there are two ways by which disturbances outside the frequency range of a circuit can interfere with it: rectification (sometimes called demodulation) and intermodulation.
All semiconductors respond non-linearly to voltages and currents, with some responding more linearly than others. The typical semiconductor is often assumed to have a square-law response at low levels of current, although this is often a very crude assumption. The effect of passing a voltage or current through a semiconductor is that positive-going waveforms are amplified more than negative-going (or vice-versa, depending on the polarity of the device) – resulting in a signal-dependent d.c. offset, known as signal ‘rectification’. When an RF signal passes through a semiconductor, modulation of the RF signal causes the d.c. offset to vary accordingly. This effect is used in radio receivers to demodulate the RF signal, but it occurs in all semiconductors (including low-frequency operational amplifiers used for audio and instrumentation), so all semiconductors should be thought of as ‘RF detectors’, essentially little radio receivers.
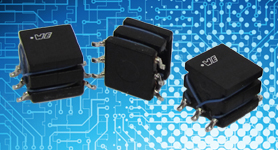
When more than one RF noise signal is present at the same time, non-linearities create ‘intermodulation products’. The presence of f1 together with f2 (say 100 MHz and 100.02 MHz, typical of two adjacent FM broadcast channels) will result in intermodulation products at f1 – f2 (20 kHz) and at f1 + f2 (200.02 MHz). Three initial frequencies create eight intermodulation products in total, and with four and more initial frequencies the situation is even more complex. The simple sum and difference frequencies between pairs of initial frequencies can have significantly high levels in some circumstances, leading to the situation where it is the intermodulation product that causes the equipment to fail.
All transistors are semiconductors, and are used in all analogue and digital integrated circuits as well as in discrete devices (e.g. power transistors). But many other types of devices are also semiconductors, for example: diodes, rectifiers, thermistors (NTC and PTC), and many types of overvoltage protection devices. Metalwork can also create ‘unintentional semiconductors’ when corrosion causes a film of oxide to form at joints. This can lead to some very unexpected real life problems; such as the Saturn launch vehicle Range Safety concerns described in ‘Banana Skin’ No. 267 [8].
Different Versions of EN 55022
The 1998 version of EN 55022 was originally intended to replace the 1994 version in August 2001, but it added a new conducted test for telecommunications cables that caused a great deal of debate, exacerbated by an error in the standard that meant that many of the new telecom’s test transducers had an error of 10dB. So it was decided to delay the DOW (the “Date Of Withdrawal”, the date on which a new standard totally replaces an old one) by two years, until August 2003.
When the new DOW of August 2003 loomed the debate over the new tests was still not resolved, so another delay of two years was agreed. The 1998 version of EN 55022 then had a DOW of 1st August 2005, but even was further delayed, and in 2006 EN 55022:2006 was adopted and became effective in October 2009. Currently, EN 55022:2010 is in the works with a DOW of December 2013. This handbook is based on the 1994 version and its amendments.
As was mentioned above, over the years there have been numerous versions of CISPR 22 and EN 55022, each with their own modifications, and sometimes the EN standards have modifications that make them a little different from their CISPR originals. When non-European countries adopt CISPR 22 for use with their own EMC regulations, they sometimes make small modifications too.
Testing with different versions of the standards can give different results, so it is important to be sure that you are applying the correct version. If you are only supplying a product into the EU, the situation is clear enough and the version of the standard and its amendments that should be used to satisfy the conformity assessment requirements is described in the official list of standards that can be reached via the website mentioned at [3].
But when supplying the same product to a number of countries or trading blocs, of which the EU may only be one, they will probably require testing to be done to a different version of CISPR 22, with different Amendments and maybe some of their own modifications. To save repeating the same tests over and over, with slight variations each time, some test labs are able to combine a number of national requirements into one test that, although longer than one test to CISPR 22 or EN 55022, saves time and cost overall. These test labs can often, at extra cost, provide a special certificate (e.g. the IEC’s “CB Scheme”) that is acceptable in many countries, supposedly avoiding the need to have their own national test labs retest your product.
When EN 55022 (or EN 55011) are Insufficient in Real Life
The problem that the emissions limits set by EN 55022 (or EN 55011) could be too high for the normal use of a type of equipment, so that it creates interference, was mentioned above in connection with the legal issues of complying with the EMCD’s Protection Requirements (Article 4 of [3]). Neither of these standards addresses the situation where sensitive electrical or electronic equipment (e.g. radio receivers, scientific instrumentation, etc.) could be used nearby. The emissions limits in EN 55022 were chosen to protect broadcast receivers whose antennas are at least 10 metres away from the equipment being tested, and even then they are not low enough to guarantee protection. In the case of EN 55011 this ‘protection distance’
is 30m.
If a product causes interference in real life, it will annoy people and can harm the future prospects of its manufacturer. Where a safety-related or mission-critical system is interfered with, people could suffer more than mere annoyance, and (in the EU) claims under the Product Liability Directive and/or Health and Safety at Work Directives could be a possible result.
Where it is wished to avoid such potential situations, designers should assess the likely proximity of their new product to sensitive equipment, or equipment whose reliability is paramount. From this will come a conducted emissions specification for the new product, usually based on EN 55022 (or EN 55011) but with lower emissions limits for some frequency ranges and/or a tested frequency range that extends lower than 150 kHz and/or higher than 30 MHz. They should then design and test their product to comply with that new specification. This will help ensure compliance with the EMCD’s Protection Requirements as well as helping to maintain good customer perceptions and reduce financial risks.
Transducers for Conducted Emissions Tests
A wide variety of transducers are available for diagnostic, development and QA measurements of conducted RF emissions, for example close-field probes and current probes, and these are discussed in [9] and [10]. Many of these can be made at low-cost using readily available components, and can provide very useful information throughout a project.
LISNs, AMNs and V-Networks
Full-compliance EN 55022 and EN 55011 tests for conducted emissions use Line Impedance Stabilization Networks (LISNs) – sometimes called ‘Artificial Mains Networks’ (AMNs) or ‘V-Networks’, and these are the standard transducer for measuring conducted emissions on the mains lead in a great many test standards. They are invasive transducers that must be connected in series with the conductors being tested to standardize their CM and DM impedances. Reference [11] is a detailed analysis of LISNs and their application.
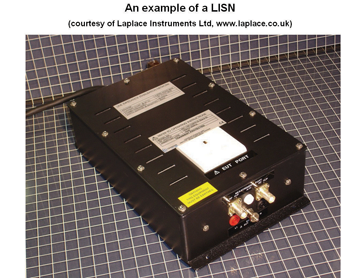
A LISN couples the interference emitted by the equipment under test’s (EUT’s) AC supply connection (mains port) to the measuring instrument (spectrum analyzer or EMC receiver). It also presents a stable and well-defined impedance to the EUT across the desired frequency range. The actual measured voltage depends on the ratio of the EUT’s source impedance and the LISN’s load impedance, so if the impedance were not stabilized, there would be no repeatability between different test locations. CISPR 16 defines the LISN and its impedance for some different classes of network: the most common one is known as the “50Ω/50µH+5Ω” network, since the impedance curve is determined by these values. A “50Ω/5µH+1Ω” network is sometimes specified where currents are very high.
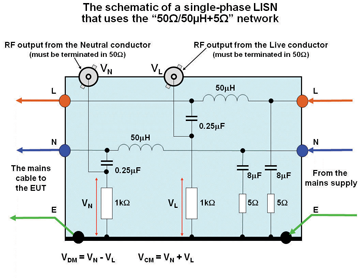
1kΩ resistors are used to discharge the 0.25µF voltage coupling capacitors when no external termination is fitted, helping to prevent damage to sensitive RF ‘front ends’. The use of transient limiters (see section 2.1.9 in [9]) should protect against such damage, but 1kΩ resistors are cheap and the RF front ends expensive so why take the risk.
The frequency response of the 50Ω/50µH+5Ω network is defined down to 9 kHz, and a fully compliant LISN will stay within the specified tolerance of ±20% of this curve whatever the termination at the mains side of the unit. This is easily achieved across most of the frequency range, but poorly designed units can exceed the specification below 50 kHz or above 25 MHz.
Like all EMC transducers, LISNs must be calibrated and their calibration factors (sometimes called transducer factors) taken into account whenever they are used in an accurate measurement of conducted emissions. Professional emissions measuring instruments usually incorporate algorithms that take all the calibration factors into account, so that their screen display and printouts show the accurate emissions levels. This feature makes it possible to show the pass/fail limit line on the instrument’s screen along with the corrected data, saving a great deal of time in development and diagnostic testing. (Of course, the calibration data for the transducers (such as LISNs and antennas) and cables to be used must first be entered into the instrument’s memory.)
The LISN’s earth is connected to the ground reference plane (GRP) of the test set-up. Since this is the ground reference for the measurement, no extra RF impedance should be introduced by this connection because it would affect both the impedance seen by the EUT and the voltage developed across the LISN’s impedance. This means that wires or straps of more than a few inches must not be used, since their inductance is unacceptable. The best connection here is a solid copper or brass bracket, firmly bonding the LISN to the GRP.
Perfectly good LISNs are available for purchase, but if you wish to construct your own you should recognise that constructing an accurate LISN is not as simple as its schematic might lead you to believe. Here are a few essential construction tips extracted from [11] and [12]:
- For the 50µH inductor, follow the construction described by [12].
- Use a number of large-value safety-rated capacitors (preferably Y1 rated) in parallel for the 8µF parts, and low-inductance resistors (not wire-wound types) for the 5Ω. Keep all lead lengths very short and use the chassis of the unit as the earth connection for the schematic, rather than any earth wires. Any unavoidable wired earth connections (e.g. from the earth pin of the EUT mains socket to the chassis must be no longer than 50mm and should use braid straps or metal plates at least 10mm wide.
- For the general assembly of the LISN follow [10].
Ferrite-cored inductors are available which are much smaller than the air-cored components described above and permit the construction of very compact LISNs. But when using a ferrite-cored inductor for a LISN, bear in mind that most AC-DC mains power converters do not draw sine-wave currents and if the size of the core is inadequate it may saturate at the current peaks and give incorrect results.
Commercially available LISNs may be fitted with ‘added value’ enhancements to the basic circuit, such as additional low-pass filtering for the incoming mains, and high-pass filtering for the RF output to remove most of the mains frequency.
LISNs create an artificial supply impedance of 50Ω from each line to earth making the common-mode (CM) impedance 50Ω and the differential-mode (DM) impedance 100Ω, both across a wide range of frequencies, whereas real mains supplies have CM and DM impedances that vary from 2Ω to 2000Ω (at least) depending upon the mode, the time of day, and the frequency (above 10 kHz). This means that filters that give good results on conducted emissions tests might not provide the same degree of filtering in real applications. For more on the problems of source and load impedances for filters, and on how to choose filters that are more likely to provide reliable attenuation both on LISN tests and with a very wide variety of real-life impedances, refer to section 3.8 of [13], section 8.1.3.1 of [14] or Part 3 of [15].
A very important Safety Note for all LISNs, AMNs and V-Networks
Because of the high levels of continuous earth-leakage currents associated with these devices, residual current circuit breakers (RCCBs) and other types of earth-leakage protection devices can’t be used.
So all LISNs, AMNs or V-networks must have two independent protective (safety) earth connections in place before mains voltage is applied to them. Each protective (safety) earth connection must be rated for the full value of the possible fault current (i.e. the maximum current if the live supply should short-circuit directly to the chassis).
Staff training in the safe and correct use of LISNs is strongly recommended, and untrained people and third parties must not be allowed to be within reach of LISNs or equipment or cables connected to them. A regular and documented safety check of the integrity of both the protective earth bonds is also recommended.
Warning labels on LISNs are also strongly recommended for:
- Their lethally high earth-leakage current
- The need to maintain two independent protective earth connections at all times
- Their use only by authorized and trained personnel
If a purchased (or home-made) LISN does not have the necessary warning labels already fitted, add them immediately – and in very conspicuous places.
When designing or selecting mains filters it often helps to know the levels and frequencies of the CM and DM emissions from an item of equipment. The RF outputs from a LISN are neither – they are a mixture of CM and DM – but [16] describes how a simple transformer wound on a ferrite toroid or ‘pot core’ can provide both CM and DM signals from a LISN’s outputs.
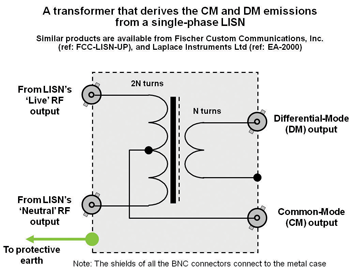
Transient limiters
Transient limiters are employed between the LISN’s RF outputs and the measuring instrument to protect the instrument’s sensitive RF ‘front-end’ from mains transients. The LISN itself will attenuate most of the transients on the mains supply – the real problem is the connection and disconnection of the EUT, which can cause 400V transients to appear at the RF outputs.
Limiters usually consist of a -10dB attenuator plus a pair of back-to-back small-signal diodes to clip the maximum signal to about ±1V. They should be calibrated, and their ‘correction factors’ taken into account along with the LISN’s own transducer factors and any correction factors for the cables, on every measurement where they are used.
(Some EMC receivers or spectrum analyzers have built-in transient limiters, in which case they are calibrated when the test instrument is calibrated and can be ignored.)
Voltage probe
CISPR , ANSI, and the FCC all describe a voltage probe that may be used to measure the conducted emissions on power terminals, usually when LISNs aren’t available or aren’t appropriate, often due to the high currents involved. But neither EN 55022 nor EN 55011 permit the use of a voltage probe, so where it is desired to use such a probe instead of a LISN it will not be possible to apply either standard in full – so for EMC Directive compliance, this would mean following the ‘TCF route’ instead of self-declaration.
Safety Note
To help prevent damage to the measuring equipment the capacitor should be a class Y type, and to prevent electric shock the probe and its output should be suitably insulated and designed with a handle that keeps its user’s fingers protected from the terminal being probed.
Measuring Instruments for Conducted RF Emissions
Instrumentation requirements for full compliance conducted emissions tests
There are two main types of interference measuring instruments: ‘EMC Receivers’ (usually just ‘Receivers’) and ‘EMC Spectrum Analyzers’ and they must both comply fully with the provisions of CISPR 16-1 if they are to be used for full compliance measurements. Instruments sold as “pre-compliance”, or without any claims regarding compliance, cost less (of course) and do not fully comply with all aspects of CISPR 16-1, so may give erroneous measurements under some conditions. To understand the issues it is worth a brief look at the constraints imposed by the specifications of CISPR 16-1. These are:
- Bandwidths
- Detectors
- Overload performance and pulse accuracy
- Input VSWR and sensitivity
Bandwidths
If an interference signal spectrum is wider than the bandwidth of the instrument that measures it, then the indicated value will depend on that bandwidth. If it is narrower, then the indicated value is independent of bandwidth. This is the basis of the distinction between “narrowband” and “broadband” interference. If you are measuring known radio signals then you can tailor the measurement bandwidth to the characteristics of the signal, but this is not possible for EMC measurements, since the characteristic of the interference is almost by definition not known in advance. Therefore the CISPR 16-1 specification includes defined values, not only for the bandwidths required for each measuring frequency range, but also for the shape of the filters that are used to provide these bandwidths.
Only a receiver whose bandwidth characteristics fully comply with this specification should be used for full compliance measurements. However, if you are only measuring narrowband interference, such as individual emissions from the harmonics of microprocessor clocks, the actual performance of the receiver filters will have little or no effect on the outcome. This is the root of much of the confusion over bandwidth requirements.
Detectors
CISPR 16-1 specifies three principal detector types: peak (PK); quasi-peak (QP) and average (AV). Most initial measurements are made with the PK detector, which responds in about 1µs to the peak value of the interference signal. Conducted emissions limits are specified by standards using the QP detector, although some ETSI emissions standards use the PK detector instead. (The AV detector is only used for conducted emissions.) Full compliance measurements must use only the correct detectors. The difference between the detectors resides in how they respond to pulsed or modulated signals. All three types of detector give the same response to unmodulated, continuous signals.
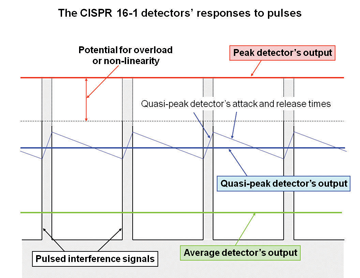
The QP detector weights the indicated value in terms of its perceived “annoyance factor”: low pulse repetition frequencies (PRF) are less annoying when experienced on broadcast radio and TV channels than high PRFs. The detector is specified in terms of its attack and decay time constants, and these are fairly straightforward to implement. The average detector simply returns the average value rather than the peak value of the interference with which it is presented. This can, in principle, be achieved with a simple low-pass filter whose time constant is slower than the slowest pulse repetition frequency of the input.
Overload performance and pulse accuracy
The difficulty which faces designers of measuring receivers is that to give an accurate measure of the actual quasi-peak or average level of interference with a low pulse repetition frequency, the linear dynamic range of the RF circuits before the detector, and of the detector itself, must be at least equal to the dynamic range of the desired pulse if they are not to be compressed and the test results made erroneous.
This dynamic range (according to the CISPR 16-1 specification) can approach 43dB, which means closer to 60dB in the receiver design. If the measuring equipment does not adjust its gain continually to achieve the optimum level at the detector – spectrum analyzers, for instance, can’t do this – then the needed dynamic range is several tens of dB more. This is a serious design challenge for RF circuits, and as a result linearity and overload performance for pulsed signals are the most important factors that distinguish low-cost and ‘pre-compliance’ test instruments from fully compliant measuring receivers.
Overloading the transient limiter with out of band signals
There is a problem with potential overload of the transient limiter. Because there is no tuned circuit before it, it can be overloaded by a powerful signal, even when that signal is far outside the frequency range being measured. The solution is to make a measurement and record it, then connect a 10dB through-line 50Ω attenuator between the transducer (e.g. a LISN) and the transient limiter’s RF input, re-measure, and compare with the original measurement.
If all the displayed signals are reduced by nearly 10dB (ignoring those that fall beneath the noise floor), then the transient limiter is not being overloaded. Any signals that reduce by significantly more than 10dB are probably intermodulation products caused by overload from a signal that is not within the measured bandwidth.
The usual way to deal with this is to determine the frequency causing the overload and to “notch it out” by adding a tunable (calibrated) notch filter ahead of the transient limiter.
Of course, if the signal causing the overload is within the measured bandwidth, then the test result is a Fail and there is no need to use a notch filter!
Input VSWR and sensitivity
Of somewhat lesser importance, but still part of the CISPR 16-1 spec, is the requirement on input VSWR (Voltage Standing Wave Ratio). This is specified to be 2:1 with no input attenuation, dropping to 1.2:1 with 10dB attenuation. VSWR is directly related to measurement error due to mismatch. For a broad-spectrum receiver (remember, 9 kHz to 30 MHz is more than three decades) the spec of 2:1 without attenuation is quite hard to meet – many ordinary radio receivers can’t achieve this even over a narrow range. It’s easy enough if you allow yourself some attenuation at the input, but then the receiver sensitivity is degraded.
The sensitivity requirement in CISPR 16-1 is expressed in the form that the noise component should not degrade the measurement accuracy by more than 1dB. This implies that the system noise floor must be at least 6dB below the lowest level it is desired to measure accurately. The system noise floor is the receiver noise plus the losses imposed by the transducer or antenna factor and connecting cables. In practice, the limiting performance is usually found at the top end of the conducted test around 1GHz. Low-cost receivers and antennas are often found to have inadequate sensitivity at these frequencies even to measure at the limit line, let alone below it.
In summary, there are several reasons for the high price of fully compliant measuring receivers, and the stringency of the CISPR 16-1 specification is a direct result of the need to make accurate measurements of an unknown and variable interfering signal, over an extremely wide range of the spectrum. Any “pre-compliance” instrument will necessarily compromise some or all of these aims.
EMC Spectrum Analyzers
There are a number of manufacturers of spectrum analyzers, some of them aiming directly at the low-cost EMC testing market. Very low-cost analyzers are also low on features and functionality, and almost nothing about them will comply with CISPR 16-1, but they are useful for development, diagnostic and QA work [9]. A good quality portable spectrum analyzer is a very useful EMC tool for a wide variety of tasks including pre-compliance testing but will almost certainly not fully comply with CISPR 16-1. It will save a great deal of time and effort if your analyzer provides automatic compensation for cable and antenna factors, scales in dBµV, shows limit lines, has reasonably accurate quasi-peak and average detectors and can save results to disc or send them to a printer.
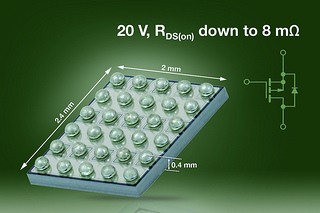
Spectrum analyzers can be overloaded by strong signals, even if they are outside the frequency band being measured, so a purist (or EMC laboratory accreditation assessor) will say that they should be used with what is known as a ‘preselector’ (basically a band-pass RF filter). Whilst most test laboratories will accept the added expense, it is often unnecessary for an unaccredited test facility unless the products being tested have high levels of pulsed or continuous emissions (as some ‘ISM’ equipment covered by EN 55011 does), or if there are some very powerful ambient signals.
To discover whether a spectrum analyzer is being overloaded is easy: use the same “external through-line attenuator method” as was described earlier for determining whether a transient limiter is being overloaded. Some analyzers are much more resistant to overload than others, and some warn the user if they detect overload. But note that an analyzer might not be overloaded even when the transient limiter is.
EMC Receivers
Spectrum analyzers have a significant edge over receivers for everyday use in development, diagnostics, and QA, because it is easier to use them to quickly get a visual display of what is going on. But it can be argued that the very best EMC measurements require a receiver rather than a spectrum analyzer, to achieve the best noise floor (dynamic range), freedom from overload, and most accurate detectors, even if compared to a spectrum analyzer that is used with a preselector. But at the expensive end of the market, both receivers and spectrum analyzers are so good these days that the difference is only of importance to world-class EMC test laboratories, or to those measuring emissions to automotive equipment standards that have some very low emissions limits (hardly any higher than the noise floor of the best available equipment).
Traditional emissions measuring receivers used to only have a tuning dial and a signal level meter, but most are now available with built-in spectrum displays or else can be connected to computers running application software that provides a spectrum display, and some can be used in ‘spectrum analyzer mode’ for faster measurements.
References
- CISPR 22:1993, “Limits and methods of measurement of radio disturbance characteristics of information technology equipment” (Note: Amendment A1:1995 and Amendment A2:1996 both apply.)
- EN 55022:1994, “Limits and methods of measurement of radio disturbance characteristics of information technology equipment” (Note: Amendment A1:1995 and Amendment A2:1997 both apply.)
- European Union Directive 89/336/EEC (as amended) on Electromagnetic Compatibility. The Directive’s official EU homepage includes a downloadable version of the EMC Directive; a table of all the EN standards listed under the Directive; a guidance document on how to apply the Directive; lists of appointed EMC Competent Bodies; and progress on the 2nd Edition EMC Directive; all at: http://europa.eu.int/comm/enterprise/electr_equipment/emc/index.htm.
- CISPR 11:1997, “Industrial, scientific and medical (ISM) radiofrequency equipment — Radio disturbance characteristics — Limits and methods of measurement” (Note: Amendment A1:1999 applies and Amendment A2:2002 is available for use now and must be applied from 1st October 2005.)
- EN 55011:1998, “Industrial, scientific and medical (ISM) radiofrequency equipment — Radio disturbance characteristics — Limits and methods of measurement” (Note: Amendment A1:1999 applies and Amendment A2:2002 is available for use now and must be applied from 1st October 2005.)
- The IEE’s 2000 guide: “EMC & Functional Safety”, can be downloaded as a ‘Core’ document plus nine ‘Industry Annexes’ from http://www.iee.org/Policy/Areas/Emc/index.cfm. It is recommended that everyone downloads the Core document and at least reads its first few pages. Complying with this IEE guide could reduce exposure to liability claims.
- “EMC-related Functional Safety – An Update”, Keith Armstrong, EMC & Compliance Journal, Issue 44, January 2003, pp 24-30, at: http://www.compliance-club.com/KeithArmstrongPortfolio.
- Many examples of interference can be found in the “Banana Skins compendium”, via a link from www.compliance-club.com or at: http://www.compliance-club.com.
- “EMC Testing Part 2 – Conducted Emissions”, Tim Williams and Keith Armstrong, EMC & Compliance Journal April 2001, http://www.compliance-club.com/KeithArmstrongPortfolio.
- “EMC Testing Part 1 – Radiated Emissions”, Tim Williams and Keith Armstrong, EMC & Compliance Journal Feb 2001, http://www.compliance-club.com/KeithArmstrongPortfolio.
- “Calibration and use of artificial mains networks and absorbing clamps (Application of transducers for CISPR -based emissions measurements)” Tim Williams and Geoff Orford, DTI-NMSPU Project FF2.6 report, April 1999. May be available from the EMCTLA: http://www.emctla.co.uk.
- “Proposed new CSA standard C108.1.5, Line Impedance Stabilizing Network (LISN)” Canadian Standards Authority (CSA), C108.1.5, 4th draft, June 1984.
- “Design techniques for EMC – Part 3: Filters and surge protection devices” Keith Armstrong, the UK EMC Journal, June 1999, pages 9-15, http://www.compliance-club.com/KeithArmstrongPortfolio.
- “EMC for systems and installations” Tim Williams and Keith Armstrong, Newnes, January 2000, ISBN 0-7506-4167-3 (available from RS and Farnell).
- “EMC for Systems and Installations”, Keith Armstrong, EMC + Compliance Journal, A series of six articles during 2000, http://www.compliance-club.com/KeithArmstrongPortfolio.
- “An alternate, complementary method for characterizing EMI filters” Michel Mardiguian and Joel Raimbourg, IEEE EMC Symposium, Seattle 1999, Volume 2, pages 882-886.
- “Making radiated and conducted compliance measurements with EMI receivers” Application Note 1302, Agilent Technologies (previously Hewlett Packard).
Acknowledgments
Some of this material was previously published in the EMC Compliance Journal’s series “EMC Testing”: http://www.compliance-club.com or http://www.compliance-club.com/KeithArmstrongPortfolio. Many thanks are due to Tim Williams of Elmac Services: http://www.elmac.co.uk; timw@elmac.co.uk, my co-author for that series.