How to Terminate Multiple Shields in a Cable Bundle
In Part 1 of this article, I shared with you the origins of my journey to assess the shielding effectiveness (SE) of screened1 cables and discussed some basic rules for terminating cable shields. In Part 2, I’ll summarize the testing I recently conducted on various approaches to improving the shielding effectiveness of screened cables used in high-frequency applications and the results from that testing.
1 In the context of this article, the words: screened; screen, or screening may be replaced by shielded; shield, or shielding respectively, and vice-versa, without any changes in meanings.
The Measured Cables
Note: all these cables’ overbraids, whether single or double layers, used the same type of braid clamped to the backshells in the same way at both ends.
Cable 1: the “Null” cable for noise floor verification (see Figure 4)
A single overbraid on its own, to check that the noise floor of the test is low enough.
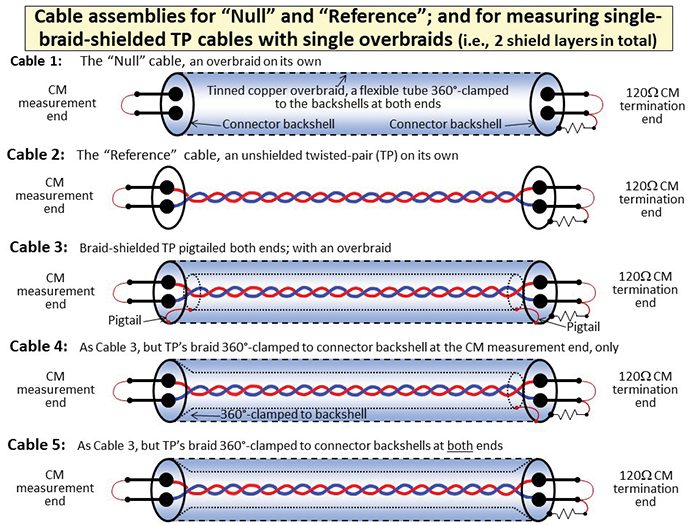
Cable 2: the “Reference” unshielded TP cable (see Figure 4)
An unshielded twisted-pair (TP) cable on its own (actually, the single-braid-shielded TP cable used to assemble cables 3 to 6, with its outer plastic jacket and shield removed).
The measured results on this cable were used as the reference that was subtracted from the measured results of each of the other cable tests (i.e., cables 3 to 12) to determine their relative SE versus frequency.
Careful control of the entire test set-up tried to ensure that the RF coupling from the antenna to the cable and the room resonance effects were identical on every test so that they canceled out. The results showed that we were reasonably successful in this.
Cables 3, 4, and 5: single-braid-shielded TP cables with single overbraids (i.e., two shield layers in total) (See Figures 4, 10, and 11)
- Cable 3: Insulated single braid TP cable pigtailed to the backshells at both ends; plus a single overbraid 360° clamped to the backshells at both ends.
- Cable 4: Same as Cable 3, but with the internal TP cable’s braid now 360° soldered to the overbraid at the backshell at the CM measured end, but still pigtailed at the 120Ω end (see Figure 7).
- Cable 5: Same as Cable 3, but with the internal TP cable’s braid now 360° soldered to the overbraid at the backshells at both ends (i.e., no pigtails at all).
Note: these cables, and Cables 6, 10, 11, and 12 below, all used the same type of single-shielded TP cable.
Cables 6, 10, 11, and 12: single-braid-shielded TP cables with double overbraids (i.e., three shield layers in total) (see Figures 5, 12, and 13)
- Cable 6: Same as Cable 3 (internal TP cable with an insulated single braid shield pigtailed to backshells at both ends), but now with double overbraids in direct electrical contact with each other along the entire cable length, and both overbraids 360° clamped together to the backshells at both ends.
- Cable 10: Same as Cable 6, but with the internal TP cable’s braid 360° soldered to both overbraids at the backshell at the CM measured end while still pigtailed at the 120Ω end (see Figure 7).
- Cable 11: Same as Cable 6 but with the internal TP cable’s braid 360° soldered to both overbraids at the backshells at both ends (i.e., no pigtails at all).
- Cable 12: Same as Cable 11, but internal TP cable’s braid exposed and making direct electrical contact with the overbraids along the entire length of the cable. That is, all three braided shields are in direct electrical contact with each other along the entire length of the cable, and all are clamped together in 360° to the backshells at both ends. This cable was very stiff!
Note: these four cables, and Cables 3, 4, and 5 above, all used the same type of single-shielded TP cable.
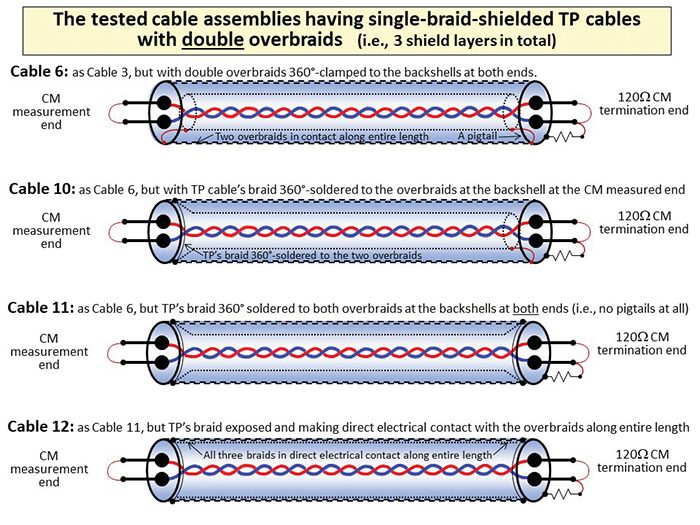
Cables 7, 8, and 9: double-braid-shielded TP cables with double overbraids (i.e., four shield layers in total) (See Figures 6, 14, and 15)
- Cable 7: Same as Cable 6, but with the internal TP cable having double braid shielding in direct electrical contact with each other along its whole length, plus an overall layer of insulation, and pigtailed to the backshells at both ends. Cable 7 also has double overbraids in direct electrical contact with each other along the entire cable, and 360° clamped together to the backshells at both ends.
- Cable 8: Same as Cable 7 but with thin mylar film inserted between the two overbraids (except where they are clamped together to the backshells at both ends).
Note: these two cables both used the same type of double-braid-shielded TP cable.

The Measurement Method
There are many ways of testing the SE of cable assemblies (i.e., cables plus their connectors), and each should be expected to give different results even with identical cable assemblies. So, I chose a test method that best represented the situation I was most interested in and that was also the easiest and quickest to do with the facilities and resources I had available at the time (see Figures 7, 8, and 9).
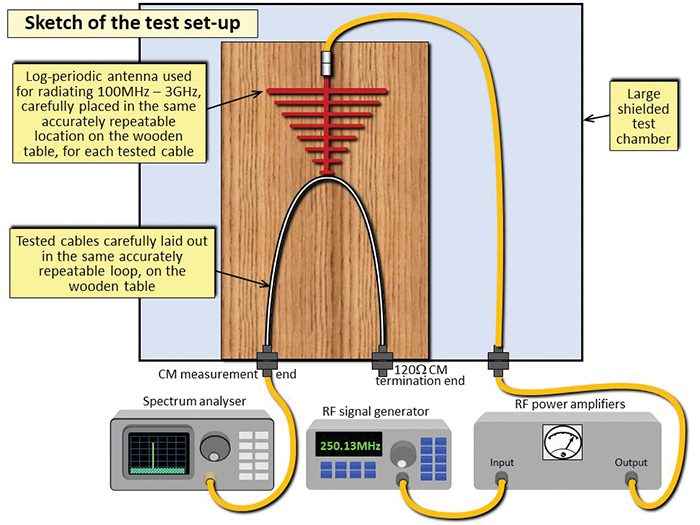


The worst of the imperfections in this method were canceled out by careful control of consistency and repeatability, and by subtracting the measured results for each cable assembly from the measurements of the Reference unshielded TP cable, Cable 2 (see above, and Figure 4).
The test chamber had once been a large TEMPEST chamber for secure communications, but for a long time had been used as a storeroom.
With a spectrum analyzer, near-field RF probe effective up to 6GHz, and a Tek box TBCG1 radiating comb generator, 100MHz – 6GHz, it did not take long to identify the RF leakages and fix them (corroded spring fingers around the door, and a telephone wire that had been brought in without RF suppression). A connector panel (visible in Figure 8) was designed, fabricated, and affixed to a hole cut in the chamber wall and also checked for RF leaks up to 6GHz.
I would have preferred either an anechoic chamber or a mode-stirred chamber, but at least the metal racking and the stored equipment in the room broke up most of its major resonant modes! And a few scraps of left-over ferrite tiles from an anechoic EMC test chamber were enough to deal with the worst remaining standing waves.
I was not interested in absolute values of SE, only in which cable design/assembly methods were the best for SE. In other words, their relative SE performances. I hoped to extract some general guidance rules for overbraid-shielded cables or cable bundles containing at least one individually shielded TP cable.
To help achieve this, with the imperfect test set-up briefly described above, a null cable (Cable 1, see Figure 4) was first measured. Being just an empty overbraid, the measurement identified any leakages from the antenna to the CM measurement pins of the bulkhead-mounted shielded connector, which included all chamber and panel leakages, and also the leakages inherent in the overbraid and its shield-bonding to the cable connectors, and from the cable connector to the bulkhead-mounted shielded connectors. This measurement showed that leakages were at or below the measurement noise floor for both frequency ranges.
Next, the Reference cable, Cable 2, was measured. This was an unshielded twisted-pair (TP) cable on its own, as shown in Figure 4, and previously described in detail.
Two different RF power amplifiers, one operating at 100MHz – 1GHz and a second at 800MHz – 2.8GHz, were used to cover the two frequency ranges reported in this article, with the above null and reference tests repeated for each amplifier.
To help achieve consistency between the different RF power amplifiers, a triaxial field probe with a fiber-optic cable passed through a waveguide-below-cutoff in the bulkhead connector panel was used to measure the field strengths around the antenna and the measured cables.
External low-noise preamplifiers with good, flat frequency responses over the measured frequency ranges were used before the spectrum analyzer’s input in cases where they would help reduce the noise floor.
All the other measured cables covered by this article consisted of the same null cable assembly used for Cable 1. Additional internal conductors and cables were made by the same very skilled cable assembler, in the same ways, with the same materials, and within a limited time span (a few days) so that we could assume consistency between them.
Given all the above and with the results from each amplifier, subtracting each cable’s results from the reference result should have substantially reduced the effects of:
- Frequency-related variations in the RF power output from each RF amplifier (see Figure 7);
- Frequency-related variations in the antenna’s response to the RF power from the power amplifiers;
- Frequency-related variations in the coupling between the antenna and the measured cables (see Figure 9);
- Frequency-related variations in the reflections from the shielded room (and the items stored in it);
- Frequency-related variations due to RF impedance mismatches in the shielded connectors, and the resulting resonances caused by the length of the cable between them; and
- There are many other possible causes of frequency-related variations in the measurements of the amplitudes of the CM noises picked up by the cables that are also reduced by the subtraction method described above, but they are all much smaller than the five listed above, so are not listed here.
This subtraction/cancellation approach was successful enough to draw conclusions on how best to terminate the shields of multiple shielded cables in an overall cable or bundle with overbraids, up to 2.8GHz. However, there were still some small errors that were deemed insignificant (see if you can spot them in the following figures!).
Results for Single-Braid-Shielded TP Cables with a Single Overbraid – Cables 3, 4, and 5
These are shown in Figure 10 for 100MHz – 1GHz, and Figure 11 for 800MHz – 2.8GHz.

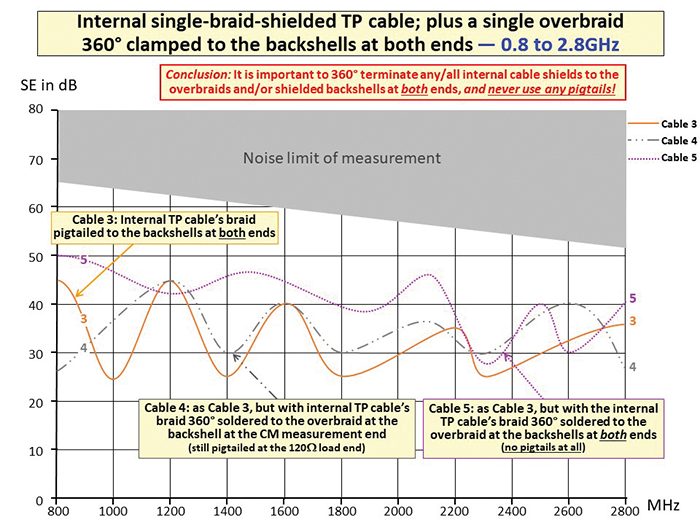
Conclusions for Single-Braid-Shielded TP cables with a Single Overbraid – Cables 3, 4, and 5
- Above 100MHz, the SE of these cables does not generally appear to continually degrade at the rate of 20dB/decade implied in Figure 1. Instead, they generally degrade more gradually and become more consistent as frequency increases. I don’t know why this was the case and will not speculate here.
- Fluctuations (frequency ripple) of up to ±12dB are seen on Cable 5, as predicted by the TRIAX BRAID curve in Figure 1, and I was pleased to have replicated it here.
Apart from the UK defense standards referenced later, most of the other documents do not mention this effect at all. In my experience, this effect is much less widely known in real-world engineering than whether shields should be terminated at one end, the other end, or both ends.
Ripples up to ±20dB are seen on Cables 4 and 3, which have pigtails at one or both ends, respectively. - It is important to 360°-terminate any/all internal cable shields to the overbraids and/or backshells at both ends – and never use any pigtails.
Results for Single-Braid-Shielded TP cables with Double Overbraids – Cables 6, 10, 11, and 12
These are shown in Figure 12 for 100MHz – 1GHz, and Figure 13 for 800MHz – 2.8GHz.


Conclusions for Single-Braid-Shielded TP Cables with Double Overbraids – Cables 6, 10, 11, and 12
- Above 100MHz, the SE of these cables does not generally appear to continually degrade at the rate of 20dB/decade implied by Figure 1. The SE of Cables 10 and 6, which have pigtails at one or both ends, respectively, degrade more gradually than this as the frequency increases.
However, Cables 11 and 12 (which have no pigtails) maintain a consistent SE up to 1GHz.
Between 1GHz and 2.8GHz, Cable 11’s SE degrades gradually as the frequency increases, but Cable 12’s SE remained so good that it was in the noise floor and we could not measure it with this test set-up in this frequency range. - Fluctuations (frequency ripple) of up to ±6dB are seen on Cable 11, a little worse than this for Cable 12.
Ripples up to ±20dB are seen on Cables 10 and 6, which have pigtails at one or both ends, respectively. - It is important to 360°-terminate any/all internal cable shields to the overbraids and/or backshells at both ends – and never use any pigtails.
Results for Double-Braid-Shielded TP Cables with Double Overbraids – Cables 7 and 8
These are shown in Figure 14 for 100MHz – 1GHz, and Figure 15 for 800MHz – 2.8GHz.

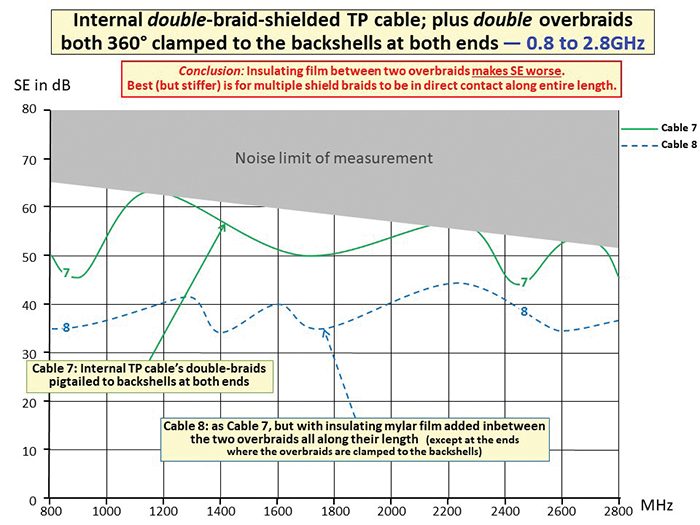
Conclusions for Double-Braid-Shielded TP Cables with Double Overbraids – Cables 7 and 8
Note: both of these cables use an internal TP cable with a double shield that is pigtailed at both ends.
- Above 100MHz, the SE of these cables does not appear to continually degrade as fast as the rate of 20dB/decade implied by Figure 1.
However, Cables 11 and 12 (which have no pigtails) maintain a consistent SE up to 1GHz.
Between 1GHz and 2.8GHz, Cable 11’s SE degrades gradually as the frequency increases, but Cable 12’s SE remained so good that it was in the noise floor and we could not measure it with this test set-up in this frequency range.
- Fluctuations (frequency ripples) of up to ±20dB are seen, which is fairly typical of all the cables that use pigtails, at one or both ends, in all these measurements.
- Adding an insulating film between two overbraids makes SE worse (not up to 30dB better, as claimed in some of the later references).
It is much better for SE (although not for mechanical flexibility) for multiple overbraids to be in direct contact along their entire length. - Comparing the measurements of Cables 7 and 8 with those of the other cables discussed in this article, we see that their rate of fall in SE as frequency increases and their frequency ripples affirm the need to 360°-terminate any/all internal cable shields to the overbraids and/or backshells at both ends – and never use any pigtails.
Final Comment
I would expect double-braid-shielded TP cables in an overall cable or bundle with double overbraids, with all shield layers 360° terminated to the overbraids and/or backshells at both ends (and no pigtails at all), to give better results than any of the cables measured above. But we did not assemble or measure such a design.
But How to Terminate the Shields of Internal Cables Without Using Pigtails?
Few publications in the public domain (including mine) address how to terminate the shields of individually shielded cables within overbraided cables or cable bundles (ignoring those recommending pigtailing through connector pins!).
This is perhaps because it tends to be an issue for high-spec military or aerospace companies, whose internal design/assembly guides often seem to me to be specifying outdated or non-cost-effective practices, such as pigtailing via connector pins, or requiring a great deal of (costly!) manual assembly by skilled personnel (e.g., 360° soldering an internal braid to an overbraid).
How to cost-effectively terminate cable shields could, on its own, easily fill a whole article, but rather than extend this article by a few thousand words I’ve added Figures 16 to 18, taken from my training course on cable EMC [25], and hope they are sufficiently self-explanatory.
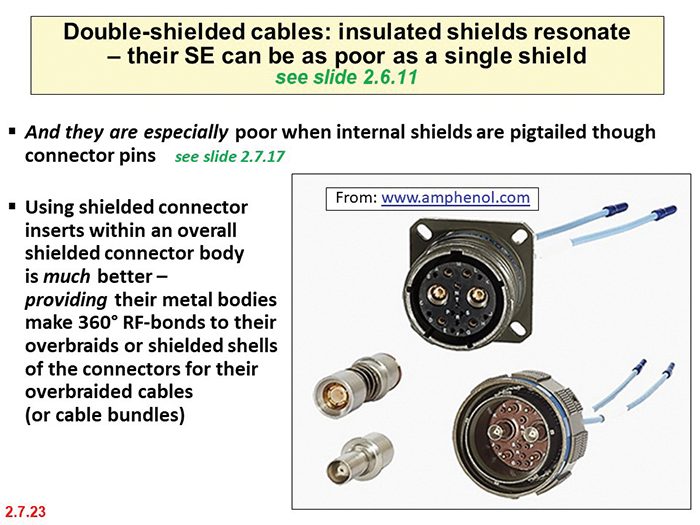
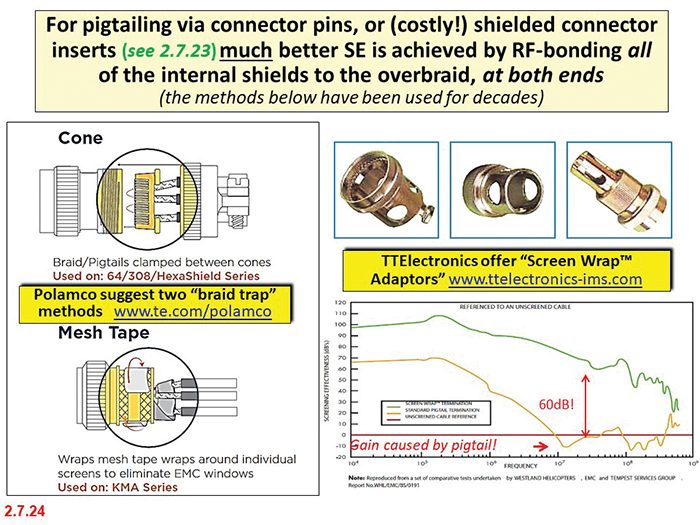
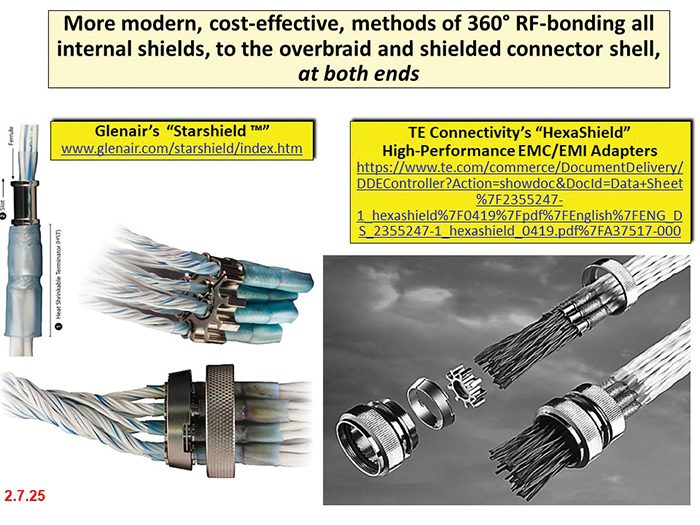
Acknowledgments
I would like to thank Lockheed Martin (UK) Ltd, near Ampthill, for the use of their facilities and for providing the test equipment used.
I would also like to thank the many people at LM(UK) who helped with these tests, particularly the following:
- Paul Moore (who made the resources available);
- Richard Clark (for helping convert his storeroom back into a shielded room);
- Chris Angove for his assistance with the shielded room and the measurements (including performing most of them and processing their data); and,
- Sean Tunn for his awesome knowledge and expertise in making shielded military cable assemblies.
References
(Note that 1 and 3 through 8 are available as free downloads from official websites)
- Ministry of Defence (UK), Defence Standard 59-411 Part 5, Issue 3, 14 June 2019, “Electromagnetic Compatibility – Part 5 : Code of Practice for Tri-Service Design and Installation.”
- All conductors (including any metalwork) are accidental antennas (whether we want them to be or not!). See https://www.emcstandards.co.uk/the-physical-basis-of-emc and/or the webinars available at https://www.emcstandards.co.uk/understanding-emc-basics-a-3-part-series.
- Ministry of Defence (UK), Defence Standard 59-41 (Part 7)/Issue 1, 10 November 1995, “Electromagnetic Compatibility – Part 7: Code of Practice for HM Ships – Installation Guidelines.”
- Ministry of Defence, Defence Standard 59-41 (Part 6)/Issue 1, 26 August 1994, “Electromagnetic Compatibility – Part 6: Code of Practice for Military Vehicles – Installation Guidelines.”
- SSP 30242 Revision E, NASA, “Space Station Cable/Wire Design and Control Requirements for Electromagnetic Compatibility – International Space Station,” Revision E, 22 December 1998.
- MIL-HDBK-1857, 27 March 1998, “Department of Defense – Handbook – Grounding, Bonding, and Shielding Design Practices.” This is an unchanged re-issue of MIL-STD-1857 (EL), dated 30 June 1976.
- MIL-STD-1310G (Navy), 28 June 1996, “Department of Defense – Standard Practice for Shipboard Bonding, Grounding, and Other Techniques for Electromagnetic Compatibility and Safety.”
- NAVAIR AD 1115, “Electromagnetic Compatibility Design Guide for Avionics and Related Ground Support Equipment,” 3rd Edition June 1988.
- IEC 61000-5-2:1997, “Electromagnetic Compatibility (EMC) – Part 5: Installation and mitigation guidelines – Section 2: Earthing and cabling,” from the BSI and IEC web shops.
- “EMC for Systems and Installations,” Tim Williams and Keith Armstrong, Newnes 2000, 0-7506-4167-3, https://www.emcstandards.co.uk/emc-for-systems-and-installations2.
- Patrick G. Andre and Kenneth Wyatt, EMI Troubleshooting Cookbook for Product Designers, Scitech Publishing, 2014, ISBNs: 978-1-61353-019-1 (hardback) 978-1-61353-041-2 (PDF), see subsections 4.8.1 and 6.8.
- Henry W. Ott, Noise Reduction Techniques in Electronic Systems, Second Edition, 1988, Wiley Interscience, ISBN 0-471-85068-3.
- William G. Duff, Designing Electronic Systems for EMC, Scitech Publishing, 2011, ISBN: 978-1-891121-42-5.
- Elya B. Joffe and Kai-Sang Lock, Grounds for Grounding, Wiley, IEEE Press, ISBN: 978-0471-66008-8.
- “Unleash the Full 5G Potential with mmWave,” https://www.qualcomm.com/research/5g/5g-nr/mmwave.
- “Fact Sheet: Spectrum Frontiers Rules Identify, Open Up Vast Amounts of New High-Band Spectrum for Next Generation (5g) Wireless Broadband,” https://docs.fcc.gov/public/attachments/DOC-340310A1.pdf.
- “There are no low-frequency systems any more!” https://www.emcstandards.co.uk/there-are-no-low-frequency-systems-any-more. Also relevant:
https://www.emcstandards.co.uk/ground-power-bounce-cause-noise-emissions-from. - “Shielding and Filtering Don’t Work Independently of Each Other,”
https://www.emcstandards.co.uk/shielding-and-filtering-don-t-work-independen. Also relevant: https://www.emcstandards.co.uk/skin-effect-and-surface-currents1. - “Saving time and money with good EMC design,” https://www.emcstandards.co.uk/saving-time-and-money-with-good-emc-design2.
- https://www.emcstandards.co.uk/testimonials-2014
https://www.emcstandards.co.uk/testimonials-2017 - “What’s in a name?” https://www.emcstandards.co.uk/what-s-in-a-name.
- “Earth Loops, Ground Loops, and Hum Loops,” https://www.emcstandards.co.uk/earth-loops-ground-loops-and-hum-loops.
- Lothar O. Hoeft and Joseph S. Hofstra, “Electromagnetic Shielding Provided by Selected DB-25 Subminiature Connectors,” IEEE 1991 International Symposium on EMC, August 12-16, 1991, Cherry Hill, NJ., ISBN: 0-7803-0158-7, https://ieeexplore.ieee.org/document/148182.
- Michel Mardiguian, “Simple Method for Predicting a Cable Shielding Factor, Based on Transfer Impedance,” Interference Technology Magazine, EMC Directory & Design Guide 2012, http://www.interferencetechnology.com/wp-content/uploads/2012/03/Mardiguian_DDG12.pdf.
- Cherry Clough Consultants’ EMC Training Course, Module 2, EMC in Cable Interconnections; course notes, https://www.emcstandards.co.uk/2-emc-in-interconnections-techniques-for-cables.