Fully anechoic rooms (or FARs) are now included as certified test sites in the generic standard CISPR 16-1-4 Ed 3.2. Although limitations exist for floor standing equipment, they significantly reduce cost and facility requirements for many products. Without a scanning mast or the need to change over floor absorber configurations, test time is reduced with improved measurement uncertainty. Currently, CISPR product standards are being revised to include these alternate test site solutions.
Historical Overview
In 1992, the first ferrite-only, 3-meter semi-anechoic chamber (SAC) was built in South Carolina, and subsequently “filed” by the U.S. Federal Communications Commission (FCC) in January 1993. The chamber met the certification requirements of the FCC rules under Parts 15 and 18. It was also compliant with the requirements of ANSI C63.4-1991 and, with the addition of a hybrid floor panel, met IEC 801-3 field uniformity requirements for radiated immunity.
The grid ferrites used in this chamber were less than one inch thick and essentially replaced the current solutions that utilized urethane absorbers of six or more feet in height. This eliminated the need for high bay facilities and was a total, cost-effective solution. Chamber suppliers quickly developed hybrid solutions, which utilized grid or flat ferrite tiles with matched absorbers to meet the evolving international standards. These absorbers primarily used either polyurethane and polystyrene materials.
The hybrid technologies and the standards developed at that time pretty much remained the same until CISPR, IEC and ANSI committees began to draft requirements in the early 2000s to meet the need to test at frequencies greater than 1 GHz. In 2012, CISPR 16-1-4 Ed 3.1 2012-07 was published. This established new requirements for measurement of emissions for the frequency range 1 GHz to 18 GHz.
In order to provide reflection free conditions for testing > 1 GHz in these SACs, absorber materials are placed on the ground plane between the measurement mast and the equipment under test (EUT). Site validation for these test facilities is performed by measurements referred to as site voltage standing-wave ratio, or Site VSWR. In effect, for testing frequencies greater than 1 GHz, the traditional SAC was modified into what might be considered a quasi-FAR.
Test Sites Without Ground Plane (FAR)
A FAR is a fully absorber lined shielded enclosure. These configurations have been used extensively in antenna and microwave ranges for military applications. However, with EMC testing, the standards typically included reflective/conductive ground planes. With a FAR, the intention is to create a free-space condition with only direct rays from the EUT or transmit antenna reaching the receive antenna.
The impetus for the committees to draft this new configuration has been the following potential benefits:
- Reduced overall chamber size as compared to equivalent path length SAC;
- Elimination of the scanning RX mast decreases chamber height and the need for facility clearance heights of 20 to 24 feet;
- Smaller chambers dramatically reduce overall acquisition costs;
- A fixed measurement height reduces test time; and
- Elimination of the reflecting ground plane improves the uncertainty budget.
As part of the new CISPR 16-1-4 document, the CISPR standards committee developed site validation methods and criteria for FARs, specifying maximum test diameters and heights for 3-, 5- and 10-meter test distances. The 5-meter test distance is now a new inclusion in the emissions standards. The FAR test volume is measured at three levels, bottom, middle and top, with a fixed position for the receive antenna (see Figure 1). The two methods for FAR validation are the reference site method (RSM) for path lengths of 3 and 5 meters, and traditional NSA for 5 and 10 meter distances. The RSM is required in the shorter paths in order to reduce coupling or near field effects related to biconical receive antennas. Note that the standard recommends against the use of the typically larger hybrid (biconical/LPDA combination) antennas in the range for 30 MHz to 1000 Mz.
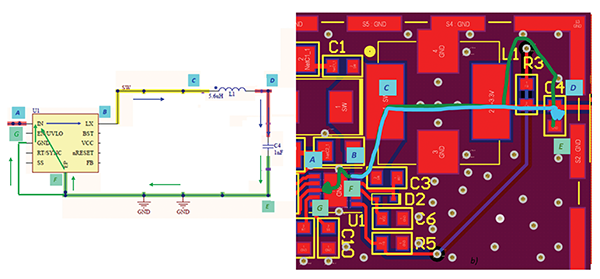
Early installations of FARs have mostly been in Europe, and are typically the 3-meter test distance configuration. In all cases, all surfaces of the shielded chambers are covered with ferrite tiles. Depending on a vendor’s hybrid absorber performance, coverage with polymaterial-based absorbers can vary from full to partial coverage.
Additional benefits from the use of FARs are that they also can meet the radiated immunity requirements of EN 61000-4-3 for a 1.5 meter by 1.5 meter uniform field plane. With a 3-meter SAC, the user needs to change the floor absorber configurations for both site VSWR and field uniformity. With the FAR, the layout is compatible for both RF immunity and emissions. This eliminates storage problems, change over time, and wear and tear on the hybrid absorbers. Last but not least, data has also been taken for compliance to the 3 meter NSA requirements of ETSI TR 102 273-2 (30 MHz -12.75 GHz).
Figure 2 shows an internal photograph of an existing site at an independent test house in France. Dimension will vary based on each vendor’s design, but typically the 3-meter FAR will be 8 meters long by 5 meters wide by 4 meters high. This is a volume difference of approximately 160 cubic meters (50 percent) as compared to a standard 3-meter SAC. The resulting cost reduction of a 3 meter FAR versus a 3-meter SAC is 25 to 35 percent, depending on options such as doors size, raised floors and power filters. External dimensions of a 5-meter FAR are approximately 12 meters long by 8 meters wide by 7 meters high.
However, the key advantage is the height difference between the FAR and the SAC, which eliminates the SAC option in many facilities. This difference would allow more companies to reduce their outside test expenses, including travel and scheduling issues for the outside vendor.
As with any radiated test chamber, performance to the certification standards is always paramount. Figures 3a and 3b show RSM performance at 3 meters in an existing FAR from 30 MHz to 1 GHz. The data reflects similar excursion patterns of that of a well-designed 3-meter SAC.
Figures 4a and 4b illustrate the results for the same chamber for the site VSWR acceptance criterion for 1 to 18 GHz. In this case, it is evident that a fully anechoic configuration provides for better performance against the 0-6 dB requirements as that of a typical SAC with partial absorber floor coverage.
Summary
The use of FARs as an alternative to traditional 3- and 5-meter semi-anechoic chamber is seeing widespread acceptance in Europe. Because of the reduced chamber height made possible by the elimination of the scanning mast in a SAC, these facilities can now be constructed in almost all manufacturing and laboratory sites. Reduced size impacts acquisition costs. The free space environment eliminates ground plane error and testing at a fixed height improves of test throughput. And the FAR is a single configuration, requiring no modifications to switch to radiated immunity and emissions testing > 1 GHz.
On the regulatory side, CISPR 16-1-4 ed3.1 is an official published document. The new pending draft CISPR 32 will allow for FARs as an alternate test site, and other product standards will follow in the future. FARs also meet EN61000-4-3 for compliant RF immunity and ETSI TR 102-273-2. One can probably safely assume that FARs will also be included in future editions of ANSI C63.4, followed by the FCC’s acceptance of FARs as alternate test sites.