How Interconnects are a Path for Potential GPR-Caused Failures
Editor’s Note—We are grateful to the family of Al Martin for giving us permission to publish this article posthumously and to honor Al and all his contributions to our industry during his long and industrious career. We would also like to thank Mick Maytum and Joe Randolph for their assistance in preparing the initial draft for publication. Thanks to you all!
Much has been written about failures due to ground potential rise (GPR). So, is there anything more to say? Well yes. What has been written has generally been about single houses with multiple grounds. But often there is not just a single house, but multiple houses all potentially interconnected via a single piece of equipment. For example, in homes connected to a distribution point unit (DPU) (see Figure 1) the DPU is often a fiber-to-the distribution point (FTTdp) unit, or a digital subscriber line access multiplexer (DSLAM). So, what happens in this case?
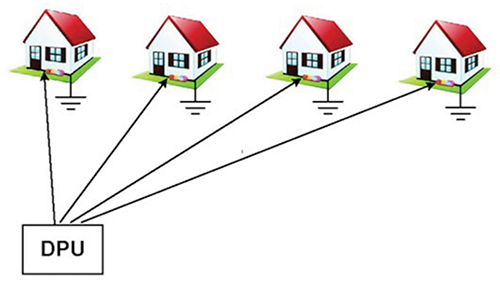
Well, what could happen is insulation failure. This kind of failure was discussed in a 2011 study by the Japanese telecommunications company Nippon Telegraph and Telephone (NTT). That company had a problem. About 0.05% of their optical network terminal/home gateway ONT/HGW boxes stopped working due to insulation failure. Now 0.05% doesn’t sound like much, but with 15 million installed devices, that amounted to 7500 units per year. Their research showed that the 0.05% failure rate corresponded to a 7.7 kV surge. Assuming that 0.05% (or less) is an acceptable failure rate, could GPR cause the corresponding 7.7 kV insulation failure?
Actual GPR measurements would give the best answer, but they don’t exist. Instead, we’ll proceed by modeling the various possibilities to see what they might predict.
Classical GPR
It may be helpful to begin with a bit of a review. In a uniform earth, a lightning strike creates a series of equipotential surfaces according to the following relation:
where ρ is the ground resistivity, I is the lightning current, and r is the distance from the lightning strike. In the uniform earth case, GPR plots in the pattern shown in Figure 2, which shows a series of equipotential surfaces of decreasing voltage (V1 > V2 > V3, etc.).
Now suppose there are grounding points A and B located on different lightning-induced equipotential surfaces like those shown in Figure 3.
From the GPR analysis, we know that the voltage at point A is greater than the voltage at point B. Thus, equipment grounded at both point A and point B can have an internal voltage difference. If the voltage difference is big enough, insulation failure and possibly destructive current flow can occur.
So, let’s see how this analysis can be extended to multiple houses, all potentially interconnected via a single piece of equipment.
Cases to Consider
To keep things manageable, consider two existing types of interconnected equipment: one in which the DPU is an FTTdp (also known as fiber to the curb, or FTTC), and the other is a DSLAM. These both use a twisted-pair POTS (plain old telephone service) line to connect the DPU to a house. The difference for GPR analysis is that for the FTTdp the maximum reach is 300 m, whereas the maximum reach of the DSLAM is 1000 m. The ground configurations can also be different.
GPR Effects for Multiple Locations with a Mutual Connection
There are six cases to consider, comprising two main cases each with three subcases:
Case 1 in which both the DPU and the houses are grounded and connected by an unshielded twisted-pair POTS line (see Figure 4). This case applies to both FTTdp and DSLAM. There are three subcases:
- Case 1a where there are no protectors installed on the POTS line
- Case 1b where one protector is installed on the POTS line
- Case 1c where there are protectors at both ends of the POTS line
Case 2 in which the houses are grounded but the DPU is not, and the DPU is connected to the houses by an unshielded twisted pair POTS line (see Figure 8). This case applies to FTTdp only.
- Case 2a where there are no protectors installed on the POTS lines
- Case 2b where one protector is installed on one of the two POTS lines
- Case 2c where protectors are installed on the POTS lines at the houses
Case 1a: The DPU and the houses are grounded and connected by an unshielded twisted pair
Figure 4 illustrates Case 1a where there is a house connected to the DPU, but there are no protectors installed on the POTS line under consideration.
In this figure:
- Rg1 is the ground resistance between the lightning strike point and the nearest ground rod (#1)
- Rg2 is the ground resistance between the farthest ground rod (#2) and infinity
- Zr1 is the impedance of ground rod #1
- Zr2 is the impedance of ground rod #2
- Rt is the resistance of the twisted pair
- Lt is the inductance of the twisted pair
- Vr1 is the voltage across ground rod #1
- Vr2 is the voltage across ground rod #2
In order to do the calculations, we need to make some assumptions. These are:
- The lightning flash is a 30 kA 4.5/75 median lightning surge from CIGRE TB549.
- The lightning flash is 50 m from the DPU.
- The maximum distance of the FTTdp from a house is 300 m
- The maximum distance of the DSL from a house is 1000 m
- The minimum distance of both FTTdp and DSL from a house is 10 m
- A 22 AWG twisted pair is the connection between the DPU and the house
- A uniform ground of resistivity ρ of 400 ohm-m (about average, according to MIL-HDBK-419).
- An 8-foot 5/8-inch diameter ground rod
The ground rod impedance Zr for calculating peak voltage was given in [2] as
where s is the length of the ground rod, a is its diameter, and d is the distance from the lightning strike to the ground rod.
From Grcev [3]:
A = αs + β (2)
α = 0.025 + exp [-0.82 (ρ ⋅ T1)0.257] (3)
β = 0.17 + exp [-0.22 (ρ ⋅ T1)0.555] (4)
From the assumptions, ρ = 400 ohm-m and T1 = 4.5 µs. For these values
α = 0.0286
β = 0.170
A = 0.240
For this case, no overvoltage protection is present either at the DPU or at the houses. Based on all the assumptions, Table 1 shows the values for the elements in Figure 4. Table 1 also shows the results of the Vr1 – Vr2 GPR calculation, run for 10 m, 50 m, 300 m, and 1000 m to cover the range of the separation distances. The peak voltage difference Vr1 – Vr2 is between the DPU and the house. The results are unchanged if more than one house is connected to the DPU.
For a median strike, TB549 Table 3.5 shows a lightning current of 30 kA, which in this case would produce the peak voltage difference shown in Table 2.
The insulation barriers shown in Figure 4 are in series, so the sum of the voltage withstand for the two barriers must be exceeded for failure to occur. Dynamically, the voltage sharing of the two barriers will also be influenced by their capacitance values. Insulation failure could be caused by punch-through at a weak spot in the insulation or by inadequate creepages and clearances, as pointed out in [6]. Punch-through might not occur until the surge voltage reaches 8 kV. So, in that event, the GPR would potentially have to exceed 16 kV for failure to occur. If inadequate creepage or clearance is the issue, a lower GPR might cause failure. If any of this happens, this case becomes Case 1c.
Case 1b: Like Case 1a, except that one protector is installed on the POTS line
This case is similar to that illustrated in Figure 4, with the addition of one protector installed on the POTS line.
The calculated results for this case are the same as those for Case 1a since current won’t flow unless the insulation barrier (shown at the DPU) is broken down and the SPD is activated. So Table 1 and Table 2 apply here. The difference between the two cases is that, in Case 1a, the surge voltage needs to overcome two insulation barriers, whereas in Case 1b, the surge voltage needs only to overcome an insulation barrier and the SPD activation voltage. So, the system failure voltage for this case is generally substantially less than for Case 1a. If the insulation barrier does fail and the SPD is activated, this case becomes Case 1c.
Case 1c: Connections similar to Figure 4, but with both SPD activated
The connections in this case are similar to the ones shown in Figure 4, but with sufficient GPR to activate both SPDs or, in Case 1a or 1b, enough GPR voltage to cause the insulation barriers to fail. Assuming that happened, the result is shown in Figure 6 as shorts. Now there is a connection from the DPU to the houses via the twisted pair, so current (ITWP) can flow between the two.
The calculated results for this case are shown in Table 3 and Table 4.
For a median strike, TB549 Table 3.5 shows a lightning current of 30 kA, which in this case would produce the peak voltage difference shown in Table 4.
Case 1c extended: Connections similar to Figure 6, but with an added house
Case 1c extended deals with connections to multiple houses, as shown in Figure 7.
In this case, there are a variety of combinations of connections between the two twisted pairs. Table 5 on page 24 shows the calculated results for the case where one run is 50 m (ITWP1), and the other run (ITWP2) can be 10TB549 Table 3.5 m (yellow), 50 m (green), 300 m (blue), and 1000 m (grey). Table 6 shows the corresponding results for a median lightning strike of 30 kA (TB549 Table 3.5). The values in Table 5 and Table 6 are less than the values in Table 3 and Table 4 due to multiple current paths in parallel.
Comparing Table 6 to Table 5, the voltages and currents drop as more houses are added. The amount by which they drop depends on the relative values for the elements in the case considered.
Case 2a: There is no ground connection at the DPU and no protectors installed on the POTS lines
There is no connection to ground at the DPU in Case 2a (see Figure 8), so the GPR effect is between houses rather than between the DPU and the house. That is because the houses are potentially connected via the DPU, as shown in Figure 9. In the DPU, the insulation barrier is between twisted pairs. Again, insulation failure could be due to punch-through, or to inadequate creepages or clearances.
As shown in Figure 9, all the insulation barriers are in series, so all would have to fail to cause a GPR problem. The GPR difference between houses can be estimated from Table 2 by subtracting the GPR voltage at one location from the GPR voltage at the other. For example, the peak GPR for a house located 300 m from the DPU is 51 kV, and the peak GPR for a house located 10 m from the DPU is 10 kV, so the difference is 41 kV. If the peak GPR voltage is big enough, all the insulation barriers fail, this case becomes Case 2c.
Case 2b: Like Case 2a, except that one protector is installed on one of the two POTS lines
This case is similar to that illustrated in Figure 9, with the addition of one protector installed on the POTS line (Figure 10). The calculated results for this case are the same as those for Case 2a since there is no GPR effect unless the insulation barriers are broken down and the SPD is activated. The GPR difference between houses can be estimated from Table 2 by subtracting the GPR voltage at one location from the GPR voltage at the other, as in Case 2a.
The difference between the two cases is that, in Case 2a, the surge voltage needs to overcome three insulation barriers, whereas in Case 2b, the surge voltage needs only to overcome two insulation barriers and the SPD activation voltage. So, the system breakdown voltage for this case is generally substantially less than for Case 2a. If the insulation barriers fail and the SPD is activated, this case becomes Case 2c.
Case 2c: Protectors are installed on the POTS lines at the house, and there is sufficient GPR to activate them
As in Case 2a, there could be a GPR difference between houses because these are potentially connected via the DPU. In Case 2c, the GPR difference between houses only needs to break down the insulation barrier between twisted pairs at the DPU. Assuming that happened and the SPDs were activated, the resulting circuit would resemble that shown in Figure 11.
A table similar to Table 5 can be constructed for Case 2c, the main difference being that there is no ground rod #1 (or at least, no connection to it). That being the case, there are again various combinations of runs of the two twisted pairs. Table 7 shows the calculated results for the case where one run is 50 m, and the other run can be 10 m (yellow), 50 m (green), 300 m (blue), and 1000 m (grey). Table 8 shows the corresponding results for a median lightning strike of 30 kA (TB549 Table 3.5).
In Table 8, as the TWP2 distance goes from 10 m to 50 m, the GPR voltage and resulting current in the twisted pair approach zero as the two locations converge on the same equipotential surface. Then, as the TWP2 distance further increases, the GPR voltage and resulting current in the twisted pair increase as the two locations diverge from the same equipotential surface.
Results and Discussion
The Results of Case 1
The results for Case 1 were shown in Table 2 and Table 4.
Looking at the tables for a 30 kA 4.5/77 lightning strike, the peak GPR could be anywhere from 1.2 kV to 66 kV, depending on the assumptions made. For Case 1c where there is a connection between the DPU and the houses, there is a current flow that could potentially be destructive.
To visualize the results, the GPR voltages in Table 2 and Table 4 are plotted in Figure 12. The initial portion of the plot for Case 1c shows the effect of the ground rod.
The Results of Case 2
A GPR effect is not likely unless the insulation barriers fail or the SPDs (if present) are activated. If that happens, then we have Case 2c (Table 8).
This case looks less severe than Case 1, but the peak GPRs could be enough to cause problems.
Observations
The results of the calculations are generally consistent with those reported by Pretorius’ study of large electrode systems [4]. For N connections, the breakdown voltage in some cases is the single connection case divided by N. The results were for median values of the variables and could be more or less depending on the assumptions made and the configuration of the connections.
Will GPR Cause Failures?
Like much in surge protection, the answer is “it depends,” and it depends first of all on what an acceptable failure rate is. The issue was cast this way by the NTT study of network equipment failure, where an 0.05% failure was too much to be acceptable. Considering that result, insulation failure in equipment could cause an unacceptable failure rate when individual insulation barriers withstand less than 8 kV. Failure would be expected if the sum of the individual insulation barrier voltage withstands and the activation voltage of any SPD present is less than the relevant peak GPR in Table 4 or Table 8. Things that could diminish the ability of an insulation barrier to withstand 16 kV include inadequate creepages and clearances [6].
Mitigation
One way to mitigate overvoltage damage is to install overvoltage protection. However, doing that might actually make the problem worse [7]. A better approach might be to increase the voltage withstand of insulation barriers where possible by installing an appropriately rated isolation transformer. This approach was done successfully by NTT [8].
Summary
So where does all this lead? The calculations are just examples. Actual GPR could be more or less, depending on the distance of the lightning flash from the DPU, the distances to the houses, the presence or absence of ground connections, and the other variables. However, the calculations suggest that GPR attributable to lightning GPR could be sufficient to overcome the insulation barrier withstand voltages, which would lead to system failure. Failure could be mitigated by isolation, the way NTT did it. SPDs could also be used, but they might not solve the problem.
References
- Martin, A. R., 2011a, “A new TIA standard for equipment installations with two or more separate grounds,” Alliance for Telecommunications Industry Solutions (ATIS) PEG Conference 2011, https://www.atis.org.
- Martin, A.R., “How Grounds Affect the Peak Voltage Due to Lightning,” In Compliance Magazine, April 2021, pp 37-40
- Grcev, L., “Impulse Efficiency of Ground Rods,” IEEE Transactions on Power Delivery, vol. 24, no. 1, January 2009, pp 441-451.
- Pretorius, P. H., “Was Lightning Ground Potential Rise Overlooked in the Design of Large Earth Electrodes?” EE Publishers, March 26, 2018.
- Tominaga, Tetsuya, “Damage to Equipment in Japan” ITU-T Study Group 05, Technical Session on Home Networks Geneva, 29/04/2011, https://www.itu.int/dms_pub/itu-t/oth/06/52/T06520000020001PPTE.ppt.
- Ardley, Tim, “Protecting PoE PSE and Ethernet to the latest international OSP standards,” The Alliance for Telecommunications Industries Solutions Protection Engineers Group, Monroe, LA, April 2016.
- Wiese, Jim, “Evolving Ethernet Applications Have Resulted in Protection Challenges,” The Alliance for Telecommunications Industries Solutions Protection Engineers Group, Littleton, CO, March 2014.
- Okugawa, Yuichiro, Honma, Yasuhiro, and Takaya, Kazuhiro, “EMC Technology that Protects Network Equipment from Electromagnetic Problems,” NTT Technical Review, March 2014, vol. 12, no. 3.