Editor’s Note: The paper on which this article is based was originally presented at the 2021 IEEE International Symposium on Product Compliance Engineering (ISPCE), conducted virtually in September 2021.
It is reprinted here with the gracious permission of the IEEE. Copyright 2021, IEEE.
Introduction
Power sources are becoming more complex as systems are devised to supply power over communication cables and other infrastructure cabling independent of the mains power supplied from the electrical grid. This document proposes to clarify how to properly test and measure the voltage, current and power with regard to these power supplies or sources that do not behave as a static mains source since the control system is embedded in the power source and includes feedback from the remote powered equipment or distribution cabling to the source to maintain proper operation, or even initiate a continuous power output.
Examples of these Intelligent or Non-Static Power Sources, include USB, Power over Ethernet (PoE), Reverse Power Feeding (RPF), and “intelligent fault managed” technologies. These technologies and Intelligent fault managed systems (sometimes called “Fault Managed Power Systems”) may include sources that sense a specified connection to some remote equipment and then turn on power, and/or, disconnects/reduces the voltage level when a fault condition is sensed. A sensed fault condition could include an abnormal load impedance or circuit, and/or a simulated human bridging.
Failure to be able to perform appropriate tests and make proper measurements based on just evaluating the source equipment can lead to unsafe equipment being placed into service and potentially create shock and fire hazards in the equipment or connected cabling.
Ease of Use
There are many things that we do from our training and experience without thinking twice about it; we call these basic assumptions and they are part of every little piece of our life. When it comes to product safety testing there is always the assumption that the needed power to run the Equipment Under Test (EUT) is readily available and comes from either DC or AC mains supplies. As such, we only reference it on the product nameplate as required by the safety standard, which is generally adequate. But we now need to examine this in more detail to properly apply the evaluation of secondary outputs from the equipment under test, called Power Sourcing Equipment (PSE), or the power into a remote device called the Powered Device (PD). The process used is well-known and typically called provisioning or configuring, which we will use in this discussion. Provisioning (typically software/firmware) or configuring (typically hardware) includes specifically providing the firmware/software plus the load equipment which needs to be adjustable for the various Normal, Abnormal, and Fault tests required. It may also be necessary to use support equipment that communicates with the PSE to generate certain outputs or signals that need to be measured.
Examining the usual situation for many appliances, office and commercial single-phase equipment that receive power from the mains, the expectation is that the power source will be available in the test lab, provide the needed nameplate voltage & current and have the capacity to handle the Normal, Abnormal and Fault currents needed for proper operation during testing. Using the raw grid power certainly will be adequate for testing such equipment; having an intermediate, adjustable power supply (local generator or variable power supply) needs more scrutiny before testing begins. None of this is even mentioned in the safety standards we normally use; it is assumed.
When a PSE product also supplies power to downstream equipment there can be difficulty understanding it fully, without making assumptions about the characteristics. When evaluating a PD, the rated input (Voltage and Current or Power) must be understood. However, for the PSE which is sourcing the power to the PD, the safety standards generally do not require any labeling of these sources. So the test lab has to determine and understand the characteristics of the source to evaluate a PD, and maybe more importantly, must understand how to ensure that the PSE output source is placed into a situation that allows the tests to be performed in a manner that replicates the intent of the safety standard. And this can involve a considerable knowledge of the equipment and include support equipment, special software/firmware, and even connection of a representative PD. These concepts may be foreign to many product safety evaluations that often include only the specific equipment submitted for evaluation. When additional equipment is needed this is often called a system-level test, and many safety standards are written based on testing a single unique piece of equipment since the equipment connected in normal use is often unknown or may not even be manufactured by the same vendor. Since non-static or intelligent sources use additional control information (at one or both ends) this must be clearly disclosed and, in most cases, proper transmit and receive modules provided just to get the circuit to operate at all. Any necessary load equipment supplied is in addition to other provisioning/configuring software/firmware equipment that may be necessary.
It should be noted that IEC 62368‑1 is specifically referred to as an equipment standard and as such many labs and manufacturers test PSE’s and PD’s independently as standalone unique entities. It is often argued that IEC 62368‑1 is not a system standard and as such equipment at the remote end (whether PSE or PD) is outside the scope of the particular evaluation. This creates quite a conundrum, as it is expected that test and load the equipment is necessary to obtain the expected conditions, but without an actual opposite end of the system or a device that is representative of the worst-case device, ( or maybe even cabling where IR losses can be a significant load), you cannot attain the desired condition for the various tests. In some cases, the manufacturer of a PSE or PD does not even manufacturer the far end equipment, making this much more difficult. One simple example is PoE where a PoE switch might be manufactured by a large switch manufacturer and it may have 4 to 96 ports, with some maximum amount of power it can source with PoE powering (for instance 800 watts total), and software controls how much wattage is distributed to cabling and PD’s (such as an IP phone, security camera, or even LED lighting) based on what devices are connected and their needed power consumption. And more often than not PD manufacturers do not make the PSE’s and visa versa.
This conundrum requires rethinking of assumptions and requirements for IEC 62368‑1; where provisioning/configuration for proper testing requires a system-level concept and setup for the purposes of evaluating a single entity within the system, and also a thorough understanding of the products operation characteristics by the test lab. Both the equipment manufacturer and the test lab must come to an agreement on the equipment provided for the test setup which will allow the full evaluation of the equipment being tested; additional modules, etc. either PSE or PDs as will be required for the evaluation.
IEC 62368‑1 as well as IEC 60950-1 and IEC 62368‑3, and most other standards assume that External Information and Communication Technology (ICT) circuits that have power sourced from the PSE to the PD are fed by circuits that output a maximum voltage into an open circuit without a load, the measurement is commonly called the open-circuit voltage. In general, under load the voltage might sag as resistance is lowered (the load on the PSE is increased), and current increases until fold-back occurs (typically with switching power sources) or maximum short circuit current is supplied (typically with linear sources). These sources might respond as expected to resistive loads at the resistance values such as 2000 ohms or 5000 ohms defined in a safety standard. These values simulate a typical human body resistance under a specific condition. To be considered safe, the source either must supply a safe touch current or an acceptably low touch voltage as defined in IEC 62368‑1 Table 4, ‘Electrical energy source limits…’ to provide electric shock protection. These values are based on IEC 60479-1 using IEC 60990 measurement techniques.
However, many newer technologies and supplies have no output (or have a very small pre-turn-on or tickling output) into resistive loads. As such, it is becoming apparent that testing is not being performed as intended which includes into normal PD’s. This includes but is not limited to determination of the source classification, which affects criteria such as insulation requirements. It also affects surface temperature measurements and others to thermal loading tests as the sources are not operating at anywhere near their maximum output.
For example, PoE outputs periodic or small output voltages looking for an impedance signature from a PD. Until it sees that handshaking signature, it will not supply normal voltage and currents to power to a PD. And into the open circuit or other conditions (such as non-capacitive loads) specified in IEC 62368‑1 the PoE PSE will only send out these small signals indefinitely.
The vast majority of test reports reviewed from labs all over the world indicate no voltage or only small voltage reported for these types of PoE External circuits. This is not unexpected since IEC 62368‑1 defines using non-capacitive loads and/or open circuits to measure or load equipment. Since these conditions do not cause proper handshaking, the source activation is not accomplished such that the circuit can be characterized or evaluated against the requirements in the safety standard. Testing of the equipment or circuit(s) under the defined load conditions cannot be performed as intended until this initial check on the circuit characteristics has been successfully completed which requires a load simulator or a representative worst-case PD and cabling.
Another example is a technology that operates well beyond RFT-V and RFT-C normally (around 330-400VDC with several thousands of effective watts) but, as IEC 62368‑1 is interpreted today, would have no output since it requires a PD that is somewhat capacitive and specially designed for that PSE. The output is just an occasional pulse into an open circuit or resistive non-capacitive load which IEC 62368‑1 specifies for testing. Again, this is a failure of providing proper configuration guidance in the standard to get the circuit up and running then characterized before testing to specific load conditions.
The details and intent are anticipated to be clarified in IEC 62368‑1 4th edition in Annex B and these same clarifications described in IEC 62368‑2 Rationale to ensure these circuits are tested and reported suitably. This will be accomplished by using a proper system level method to simulate normal operation, including the conditions assumed in IEC 62368‑1 with normally tested with open circuits and various loads, including non-capacitive loads, applied. However, a question may be asked how to do an open-circuit voltage measurement whether at the PSE source or the PD load when an added cable/load is required. The measurement may have to be made under a minimal load. Other required test cases may use specific resistances to load or measure circuits and these can trip or turn off the sources. The answers are not simple and require considerable thought during the testing process. Reasonableness must be used in performing the test to demonstrate conformity with the intent of the standard.
Outlined here is specific text proposed clause-by-clause for IEC 62368‑1 to cover the issues as discussed.
The final specific text in the standard will be adjusted, of course, dependent upon which version of the standard is being updated.
Laying Out Specific Changes Needed in IEC 62368‑1
4.1.3 Equipment design and construction
Equipment shall be so designed and constructed that, under normal operating conditions as specified in Clause B.2, abnormal operating conditions as specified in Clause B.3, and single fault conditions as specified in Clause B.4, safeguards are provided to reduce the likelihood of injury or, in the case of fire, property damage.
Parts of equipment that could cause injury shall not be accessible, and accessible parts shall not cause an injury.
Where equipment is designed such that one or more of its power sources require a specific configuration technique, a load or protocol or software to turn on the supply output, keep the supply output active or obtain the intended output voltage, current or power under normal, abnormal and single fault conditions plus a method of achieving that output shall be provided for evaluation and testing and employed.
For example, connecting intended representative worst-case loads or external powered devices, and repeating with any needed load including under fault conditions in the distribution wiring and applied loads.
This is critical for determining characteristics such as output voltage and current for ES and PS classifications, use on building and other wiring, see IEC 62368‑1, Annex Q, circuits intended for connection to building wiring, as well as proper loading for heating tests.
These examples are not necessarily all inclusive.
Compliance is checked by inspection and by the relevant tests.
5.6.4.2.3 Internal circuit as the source
Where the source is a circuit within the equipment, the protective current rating of the circuit is:
The rating of the overcurrent protective device if the current is limited by an overcurrent protective device; or
The maximum output current, if the current is limited by the source impedance of the supply. The output current is measured with any resistive load including a short-circuit measured after stabilization, usually 60 s or more after the application of the load if current is limited by impedance or the current limiting device is a fuse, a circuit breaker or a PTC device, or at longer times such as 5 s or more in other cases.
If the source is a type that does not output normal voltages/currents into a resistive load, the source needs to be connected to a terminating device/impedance that turns on the source voltage/current and creates the needed test conditions including the worst-case test conditions.
5.7 Prospective touch voltage, touch current and protective conductor current
5.7.1 General
Measurements of prospective touch voltage, touch current, and protective conductor current are made with the EUT supplied at the most unfavorable supply voltage (see B.2.3).
Activation: If a power source is of a type that requires handshaking/negotiating between a load or intelligently detects loads and as a result does not allow output of normally occurring voltages/currents into resistive loads, the source needs to be connected to a terminating device/impedance that turns on the source voltage/current and generates an output under the rated conditions specified.
6.2.2 Power source circuit classifications
6.2.2.1 General
An electric circuit is classified PS1, PS2, or PS3 based on the electrical power available to the circuit from the power source. The electrical power source classification shall be determined by measuring the maximum power under each of the following conditions:
- For load circuits: a power source under normal operating conditions as specified by the manufacturer into a worst-case fault (see 6.2.2.2);
- For power source circuits: a worst-case power source fault into the specified normal load circuit (see 6.2.2.3).
If the source or load circuit are of a type that does not allow output of normal occurring voltages/currents into resistive loads, the source needs to be connected to a activation terminating device/impedance that turns on the source voltage/current and generates the worst-case power delivery under normal and fault conditions. If measuring a load device of this type, the source is the maximum effective power that can be delivered during normal operating conditions as well as faults.
The power is measured at points X and Y in Figure 34, Power measurement for worst-case fault and Figure 35, Power measurement for worst-case power source fault.
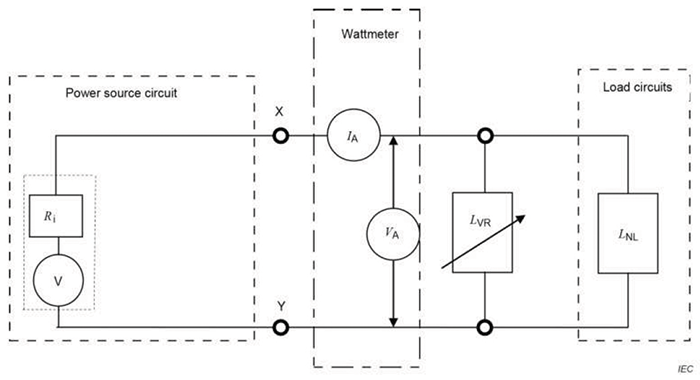
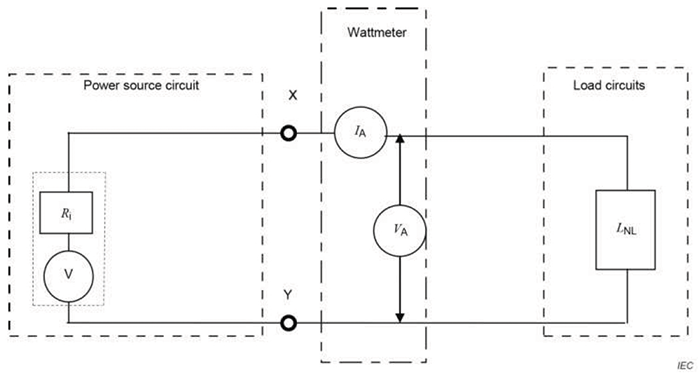
6.5.2 Requirements for interconnection to building wiring
Equipment intended to provide power over the wiring system to remote equipment shall limit the output current to a value that does not cause damage to the wiring system, due to overheating, under any normal operating conditions or external load conditions. The maximum continuous current from the equipment shall not exceed a current limit that is suitable for the minimum wire gauge specified in the equipment installation instructions.
NOTE: This wiring is not usually controlled by the equipment installation instructions, since the wiring is often installed independent of the equipment installation.
PS2 circuits or PS3 circuits that provide power and that are intended to be compatible with LPS to external circuits (see Annex Q) shall have their output power limited to values that reduce the likelihood of ignition within building wiring during normal operating and external fault conditions.
External paired conductor cable circuits, such as those described in Table 13, ‘External circuits transient voltages’, ID numbers 1 and 2 having a minimum wire diameter of 0,4 mm, shall have the current limited to 1,3 Arms or d.c.
EXAMPLE: Time/current characteristics of type gD and type gN fuses specified in IEC 60269-2 comply with the above limit. Type gD or type gN fuses rated 1 A, would meet the 1,3 A current limit.
Compliance is checked by test, inspection and where necessary by the requirements of Annex Q.
Additional Changes in the Supporting Annexes
B.3.5 Maximum load at output terminals
Output terminals of equipment supplying power to other equipment, except socket-outlets and appliance outlets directly connected to the mains, are connected to the most unfavorable load impedance, including short-circuit.
If the source is a type that does not output normal voltages/currents into a resistive load, the source needs to be connected to a terminating device/impedance that turns on the source voltage/current and creates the worst case normal and abnormal conditions.
E.1 Electrical energy source classification for audio signals When classifying audio signals as an electrical energy source (see Table E.1), the equipment shall be operated to deliver maximum non-clipped output power into its rated load impedance. The load is removed and the electrical energy source class is determined from the resulting open-circuit output voltage.
If the source is a type that does not output normal voltages/currents into a resistive load, the source needs to be connected to a terminating device/impedance that turns on the source voltage/current which creates the worst case normal and abnormal conditions.
Q1 Limited power source
Q.1.1 Requirements
A limited power source shall comply with one of the following:
- The output is inherently limited in compliance with Table Q.1; or
- Linear or non-linear impedance limits the output in compliance with Table Q.1.
If a PTC device is used, it shall:
- Pass the tests specified in Clauses 15, 17, J.15 and J.17 of IEC 60730-1:2013; or
- Meet the requirements of IEC 60730-1:2013 for a device providing Type 2.AL action;
- A regulating network limits the output in compliance with Table Q.1, both with and without a simulated single fault (see Clause B.4), in the regulating network (open circuit or short-circuit); or
- An overcurrent protective device is used and the output is limited in compliance with Table Q.2; or
- An IC current limiter complying with Clause G.9.
Where an overcurrent protective device is used, it shall be a fuse or a non-adjustable, non-autoreset, electromechanical device.
If the power source is of type that requires handshaking/negotiating between a load or intelligently detects loads and as a result does not allow output of normally occurring voltages/currents into resistive loads, the source needs to be connected to a terminating device/impedance that turns on the source voltage/current and generates the power delivery under the conditions specified.
Q.1.2 Test method and compliance criteria
Compliance is checked by inspection and measurement and, where appropriate, by examination of the manufacturer’s data for batteries. Batteries shall be fully charged when conducting the measurements for Uoc and Isc according to Table Q.1 and Table Q.2. The maximum power shall be considered, such as from a battery and from a mains circuit.
The load referenced in footnotes b and c of Table Q.1 and Table Q.2 and in Q1.1. is adjusted to develop maximum current and maximum power transfer in turn. Single fault conditions are applied in a regulating network according to Clause Q.1.1, item c) while under these maximum current and power conditions.
Output voltage a Uoc |
Output current b d Isc |
Apparent power c d S |
|
V AC | V DC | A | VA |
Uoc ≤ 30 | Uoc ≤ 30 | ≤ 8, 0 | ≤ 100 |
– | 30 < Uoc ≤ 60 | ≤ 150/Uoc | ≤ 100 |
- Uoc: Output voltage measured in accordance with B.2.3 with all load circuits disconnected. (see note e for exception) Voltages are for substantially sinusoidal AC and ripple free DC For non-sinusoidal AC and DC with ripple greater than 10 % of the peak, the peak voltage shall not exceed 42,4 V. b Isc: Maximum output current with any load, including a short-circuit.
- S (VA): Maximum output VA with any load.
- Measurement of Isc and S are made 5 s after application of the load if protection is by an electronic circuit and 60 s in case of a PTC device or in other cases. The limits are not just at 5 s and 60 s respectively, but also apply any time after that.
- For all notes in Table Q.1,
-
- If the power source is of type that requires handshaking/negotiating between a load or intelligently detects loads and as a result does not allow output of normally occurring voltages/currents into resistive loads, the source needs to be connected to a terminating device/impedance that turns on the source voltage/current and generates the power delivery under the conditions specified.
- Voltage and current are maximum ACrms, or DC peak values, Power is the effective power, and all apply regardless of frequency, or if DC is continuous or pulsed.
Table Q.1: Limits for inherently limited power sources
Output voltage a Uoc |
Output current b d Isc |
Apparent power c d S |
Current rating of overcurrent protective device e |
|
V AC | V DC | A | VA | A |
≤ 20 | ≤ 20 | ≤ 1000/Uoc | ≤ 250 | ≤ 5,0 |
20 < Uoc ≤ 30 | 20 < Uoc ≤ 30 | ≤ 100/Uoc | ||
– | 30 < Uoc ≤ 60 | ≤ 100/Uoc |
- Uoc: Output voltage measured in accordance with B.2.3 with all load circuits disconnected (see note f for exception). Voltages are for substantially sinusoidal AC and ripple free DC For non-sinusoidal AC and for DC with ripple greater than 10 % of the peak, the peak voltage shall not exceed 42,4 V.
- Isc: Maximum output current with any load, including a short-circuit, measured 60 s after application of the load. The limits are not just at 60 s, but also apply any time after that.
- S (VA): Maximum output VA with any load measured 60 s after application of the load. The limits are not just at 60 s, but also apply any time after that
- Current limiting impedances in the equipment remain in the circuit during measurement, but overcurrent protective devices are bypassed.
- The reason for making measurements with overcurrent protective devices bypassed is to determine the amount of energy that is available to cause possible overheating during the operating time of the overcurrent protective devices.
- The current ratings of overcurrent protective devices are based on fuses and circuit breakers that break the circuit within 120 s with a current equal to 210 % of the current rating specified in the table.
- For all notes in Table Q.2,
- If the power source is of type that requires handshaking/negotiating between a load or intelligently detects loads and as a result does not allow output of normally occurring voltages/currents into resistive loads, the source needs to be connected to a terminating device/impedance that turns on the source voltage/current and generates the power delivery under the conditions specified.
- Voltage and current are maximum ACrms, or DC peak values, Power is the effective power, and all apply regardless of frequency, or if DC is continuous or pulsed.
Table Q.2: Limits for power sources not inherently limited (overcurrent protective device required)
Q.2 Test for external circuits – paired conductor cable
Equipment supplying power to an external circuit paired conductor cable intended to be connected to the building wiring shall be checked as follows.
If current limiting is due to the inherent impedance of the power source, the output current into any resistive load (see note e of Q.1 or f of Q.2), including a short-circuit, is measured. The current limit shall not be exceeded any time after 60 s of test. If current limiting is provided by an overcurrent protective device having a specified time/current characteristic:
- The time/current characteristic shall show that a current equal to 110 % of the current limit will be interrupted within 60 min; and
- The output current into any resistive load, including a short- circuit, with the overcurrent protective device bypassed, measured any time after 60 s of test, shall not exceed 1 000/U where U is the output voltage measured in accordance with B.2.3 with all load circuits disconnected (see note e of Q.1 or f of Q.2).
If current limiting is provided by an overcurrent protective device that does not have a specified time/current characteristic:
- The output current into any resistive load (see note e of Q.1 or f of Q.2), including a short-circuit, shall not exceed the current limit any time after 60 s of test; and
- The output current into any resistive load (see note e of Q.1 or f of Q.2), including a short-circuit, with the overcurrent protective device bypassed, measured any time after 60 s of test, shall not exceed 1 000/U, where U is the output voltage measured in accordance with B.2.3 with all load circuits disconnected (see note e of Q.1 or f of Q.2).
Conclusions
Although conceptually straightforward, the implementation of adequate requirements to achieve the needed full evaluation of either a PSE or PD unit is complex when fitted into the overall requirements of a standard such as IEC 62368‑1 in order to ensure that they each will operate independently in a safe manner.
The details presented here are based upon personal experience in dealing with the evaluation process for this type of equipment, a detailed understanding of the requirements as laid out in the standard plus the application of reasonableness, a Hazard Based Standard adaptation technique, to the process of evaluation for these units.
The detail here will provide the user with a comprehensive roadmap through IEC 62368‑1 outlining the specific details that need to be considered in the evaluation.
Each party in the assessment process needs to apply reasonableness in adapting these evaluations, as is done in other situations (which are usually not as complex) to ensure a complete evaluation of the hazards by showing that the safeguards placed are adequate to protect the user and the local environment.
Acknowledgment
The authors thank IEC TC 108 HBSDT for feedback in dealing with this unresolved problem and to the IEC directly for base quotations from IEC 62368‑1 shown with modifications here in detail in order to deal with this ongoing difficult issue.
References
- IEC 60479 series, Effects of Electric Shock on Humans…
- IEC 60950-1, Safety of Information Technology Equipment
- IEC 60990, Measurement of Touch Current and Protective Conductor Current
- IEC 61140, Protection Against Electric Shock – Common Aspects for Installation and Equipment
- IEC 62368‑1, Safety of Information and Communication Technology Equipment