The use of exclusion zones to keep wireless transmission devices (WTDs) (e.g., radio walkie-talkies, cellular phones, etc) from being too close to instrumentation and control (I&C) equipment and system cabinets containing this equipment remains a primary concern in existing and advanced (future) nuclear power plants. In Part 1 of this article, a background and history of how exclusion zones were developed and implemented in these plants was presented along with their advantages and limitations. In Part 2, presented here, the elements of the exclusion zone strategy are discussed followed by the demonstration of a concept often used in studying the electromagnetic compatibility (EMC) immunity of modern electronic systems. This concept—called layered immunity—is key to understanding the parts of a system where EMC system immunity must be applied. The initial thought that readers must accept before proceeding to read and understand this concept described in this article is that system immunity involves other parts of the system besides just the immunity of the individual piece of equipment requiring protection.
Elements of the Current Exclusion Zone Strategy
Table 1 presents the elements of the exclusion zone strategy used today in nuclear power plants. The first element of the strategy is to require I&C equipment to have a broadband radio-frequency (RF) immunity of 10 volts per meter (V/m), consistent with the recommendations in EPRI TR-102323 [1], the NUREG 1.180[2], and International Electrotechnical Commission (IEC) recommendations for industrial equipment. To this, an 8-dB safety margin was added (see Part 1 of this article for a history of this safety margin), which results in strategy seeking to insure that I&C equipment and systems are not exposed to fields greater than 4 V/m. To ensure that I&C equipment and systems are not exposed to fields beyond 4 V/m, exclusion zones are recommended as discussed in Part 1 of this article. An exclusion zone is intended to keep wireless transceivers far enough away from I&C equipment and systems, so that their radiated emissions will not subject the I&C equipment to more than 4 V/m.
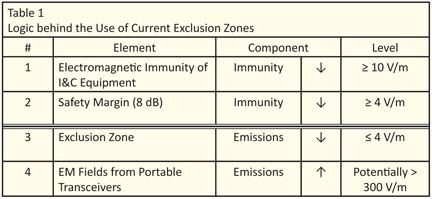
Defining Immunity Layers in Exclusion Zones – Establishing Whole-System Immunity
The concept of layered immunity to radiated emissions is not a new one in the EMC community. In many instances, it is not practical to provide all of the immunity needed in equipment design (components, circuitry, and housing) to ensure that the equipment is not affected by the emissions. Electronic equipment like that used in nuclear power plants may require solid structural mounting and protection from moisture and dust. These additional requirements require the use of a system cabinet. Traditionally, cabinets used in existing plants are not designed to provide protection against radiated threats from portable transceivers and other devices that radiate electromagnetic (EM) energy. This section describes the current exclusion zone strategy in more detail and analyzes its effectiveness.
General Approach
The electromagnetic interference (EMI) control strategy used in existing nuclear power plants assumes a two layer strategy made up of two levels. Presently, I&C systems have a level of immunity. Although that level is different for each type of I&C equipment, an assumption is being made here that it is constant. This level of immunity is the first layer. Portable transceivers have a level of EM emissions, which is the second level. An exclusion zone matches them, so that the required level of protection is maintained. In other words, the minimum distance allowed between a portable transceiver and the face of a system cabinet (defined by the exclusion zone that defines the strength of the field at the cabinet face and with the cabinet door closed) must be maintained so that the field generated by the transceiver at that distance from the transceiver’s antenna is not greater than the immunity level of the I&C equipment.
Applying the Approach to Advanced Nuclear Power Plants
For advanced nuclear power plants, this strategy will not provide the immunity protection required to avoid upsetting digital I&C equipment. Similarly, designers of advanced plants and planning engineers for digital I&C systems do not have faith in this strategy. The current strategy is insufficient because the analysis of the problem that produces it is insufficient. This reality can be quickly realized when contemplating protection from low-frequency, high-impact EM events. Portable transceivers are not the only sources of significant EM fields posing a EMI threat to digital I&C equipment. However, they are the most common source of high-field levels experienced in existing nuclear power plants. However, advanced plants require protection from not only commonly occurring events, but rarer, higher-impact events. There are, in fact, a range of more rare but high-impact events.
One example discussed in previous EPRI reports on past EMC research for nuclear power plants comes from the use of arc welding. Arc welding has been shown in existing nuclear plants to interrupt the operation of I&C equipment and systems. In many cases, when arc welding is carried out, many plant alarms are set off and indication is observed in the control room. Arc welding practices will also occur in advanced plants just like they occur in existing plants.
Arc welding is not an everyday event near most I&C systems, but it is sufficiently common, so that it receives special discussion in previous EPRI reports and special attention by nuclear plant personnel. However, when abstracted out, arc welding is simply an example of a rarer source of significant EM fields being imposed upon I&C systems. I&C engineers and maintenance personnel in nuclear plants are concerned about causing I&C equipment to trip when carrying out welding practices in the plant. Radiated and conducted threats from welding equipment frequently cause actuation of alarms and in some cases equipment trips. While efforts are made to reduce these effects by using “chicken wire” to ‘shield’ I&C equipment and careful routing of AC power and welder cables away from conduits and system cabinets, other practices may be employed to further reduce the risks of setting off nuisance alarms and tripping equipment. Past EPRI research has already determined which welders (electric, MIG, TIG, etc.) and plasma cutters cause higher levels of emissions. Upcoming near-term EPRI research efforts will seek to define new practices for controlling emissions generated by welding equipment used in power plants.
There are others. At the far end of the range would be Electromagnetic Pulse (EMP) and Intentional EMI (IEMI), introduced with the malicious intent to disrupt mission-critical equipment in various industries include I&C equipment used in nuclear and other power plants. IEMI is gaining attention in the EMC community as a serious EMC problem. Actual IEMI events have occurred and caused disruption to electronic systems. The protection plan for advanced plants must consider and address the entire range of EM events, providing life-cycle protection that is needed for new nuclear plants.
The Victim – Digital I&C Equipment and Systems
Digital I&C equipment and systems require system levels of EM immunity. Digital I&C systems are more susceptible to EM threats and thus have lower immunity. EMC researchers and digital equipment designers have known this for years. Each component in the system must participate and provide the level of immunity required of it in order to reach a total level of immunity that will ensure the proper EMC protection for the whole system and all of its peripheral devices and interfaces. Unshielded and improperly shielded power and data cables and cable feed-throughs used in connecting peripheral devices and interfaces to digital I&C equipment significantly increase the risk for EMI problems, especially in areas where portable transceivers are used. (A future IN Compliance article will address the susceptibility of digital I&C systems to EMI caused by cables and feed-throughs.) For the sake of analysis here, each layer will be assigned an immunity variable.
Dissecting the Immunity Layers inside Digital I&C Equipment
Digital I&C equipment may be considered from a layered or component viewpoint. First, there is the circuitry inside the equipment itself. This consists of the electronic passive and active components connected to the printed circuit boards (PCBs), the PCBs themselves, wiring harnesses that travel from board to board, modular backplanes (which are more common nowadays), and the peripheral devices that extend from the board through a wiring harness to the inside surface of the equipment housing (or case).
The immunity of electronic components varies according to component type (i.e., whether the component is leaded or an integrated circuit (IC) with many short leads), material used to manufacturer the components, where they are located on the PCBs among other variables. ICs may have a radiated immunity specification as well. What level of EM immunity do the electronic circuits inside the equipment have?
Second, there is the housing around each system component. Does it add to the EM immunity of the equipment? Or, does it actually decrease the immunity? Digital I&C equipment and systems have more I/O ports than other types of digital equipment used in other industries. Third, there is the system cabinet. Does it contribute and add to the immunity of the system? Again, there are multiple cable harnesses and ports that must enter and exit the boundaries of a cabinet. Each of these plays a role in establishing (or deteriorating) the overall system immunity. Forth, there are the cables going between system components inside an individual cabinet. Do they maintain the system’s immunity? Does their exposure to the EM environment inside the cabinet affect the overall immunity of the total equipment package inside the cabinet? All of these questions must be carefully answered in order to assure that the total digital I&C system provides the level of EM immunity required for digital I&C equipment and systems used in advanced nuclear plants—questions that were never answered for analog I&C equipment and systems used in existing nuclear plants. The good news is that the radiated immunity of every item mentioned above is either known or can be determined through application of standardized EMC immunity tests during the design phase of each item. Figure 1 describes the layered concept for whole-system radiated immunity.
Figure 1
The First Layer – Internal PCBs and Components
The PCBs and components inside the equipment represent the first layer. Components such as board assemblies, ICs and power supply modules can be tested for emissions and immunity as a part of the product development process before they are designed into a PCBs. Some PCBs are also tested for emissions and immunity before they are made available to equipment designers to use in equipment designs. The immunity of a populated PCB is likely to be insufficient without being mounted in an equipment assembly inside an equipment housing. EMC performance specifications can be developed for PCBs used in digital I&C equipment and systems that will define some of the basic EMC performance expected. Some of immunity weaknesses that are present in components and PCBs that cannot be resolved can be resolved through providing additional immunity from the equipment housing and providing immunity at further outer layer.
The Second Layer – OEM Equipment Housings
Figure 2 illustrates a typical electronic housing for a piece of electronic equipment. Electronic equipment is designed for use inside or outside a system cabinet. In advanced nuclear plants, digital I&C systems will be mounted inside system cabinets like they are in existing nuclear plants.
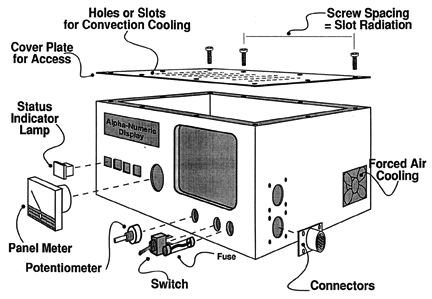
Example of Equipment Housing (Case or Enclosure) for a Piece of Electronic Equipment
Equipment housings must physically support dozens of peripheral and interface components in addition to PCB assemblies and other modules like power supplies. These components must penetrate the housing in some fashion in order to provide functionality to the user and interface to other equipment. Four types of components that are experiencing increased usage with respect to digital I&C equipment and systems are 1) light-emitting diode (LED) indicators, 2) LED displays or liquid crystal display (LCD) units, 3) I/O ports, and 4) cooling (e.g., small fans) and ventilation devices. Increased usage of these components places more demands on providing adequate immunity to EM threats external to system cabinets out on the plant floor.
Some components present more of a challenge to EMC protection (immunity) than others. Simply, the larger the surface area that is required to mount the component in the walls, top or bottom of the housing and the more electrical contact with PCBs inside the equipment the higher the concern with respect to providing adequate immunity. The following components have larger surface area
- LED display modules
- LCD modules
- Cooling fans
- D’arsonval meter movements
- Air ventilation openings
When a field strikes a component on the surface of an equipment housing, some of the EM energy is converted from radiated form to conducted form. Surface currents resulting from radiated emissions incident upon the exterior surface of a housing and upon metallic parts of penetrating components (e.g., a cooling fan) will flow on the outside surface of the housing as well as the inside surface. Those surface currents that flow on the inside surface of a housing will re-radiated to the electronic circuits inside of the housing. The amount of energy that enters the component is a function of the components geometrical design and the material from which it is made. The amount of energy that is converted from radiated form to conducted form is a function of the geometrical design of any metal parts inside the component and any PCB traces that may be present on the small boards typically part of the component (e.g., a small PCB behind an LED display module). The energy that is captured by electrical conductors (traces) is passed along to the electrical conductor (wires) that lead from the surface-mounted component on the housing down to the PCB assemblies. Some of this energy will be injected directly into the board through the wires, and some will be re-radiated out of the wires through to other components inside the equipment. Components that have more complex boards (small) and more electrical conductors entering and leaving the boards will experience more serious EM immunity issues. The following components have multiple electrical contacts to PCBs
- Key pads
- LED display units
- LCD modules
Input – output (I/O) ports are more frequently being used in digital I&C equipment just as with other digital equipment used in other industries. This increased usage stems from the ability and requirement to send more data to a digital I&C system and receive more data and from digital I&C system designs that have multiple I/O ports on their exterior panels. Equipment designers tend to include multiple I/O ports as part of their standard designs despite the fact that customers may not use them. They are also provided to enable multiple degrees of flexibility in connecting other equipment to a system. They are used for control, data communications, voice communications, peripheral (e.g., keyboards, mice, and data storage drives) communications, and other types of communications. One type of I/O port that is experiencing increased usage is the Universal Serial Bus (USB) port. Others include FireWire, miniUSB, etc.
Potentiometers, switches and even fuses are also components that penetrate equipment housings. The geometric designs of knobs and switches tend to lessen the concern regarding EM threats and immunity as those with EMC-grade specifications are available. However, their immunity should still be considered important when designing a housing and computing total housing immunity. Rotating switches may pose more of a concern if they are multi-layered and have multiple electrical conductors extending to a PCB.
Coaxial connectors are also used on some types of digital I&C equipment. Coaxial connectors range in designs and styles. Many types of coaxial connectors have EMC-grade specifications as well. Female connectors are typically used on equipment housings. Some I&C systems have multiple connectors. Some connectors will pose more of a concern to EM threats depending upon the design of the center post, dielectric, and shell that establishes the interface connection to the mating part of the connector.
“Leaks” in the Second Layer
EMC tests carried out on equipment housings demonstrate that components that penetrate the boundary of an equipment housing do decrease the immunity of the equipment. For this reason, EMC-rated components have been designed for all component categories. EMC-rated LED displays, LDC modules, I/O connector ports, switches, potentiometers, cooling fans, ventilation panels and line cord adapters are now readily available for use by equipment designers. Does the equipment designer know they are available? Does the equipment designer know that they should be designed in to digital I&C equipment to preserve and enhance EMC immunity. Yes, they will add cost to the design and testing of the equipment. However, the end result financially outweighs the cost of not including them in any digital I&C equipment design.
Significant immunity ‘leaks’ will occur in equipment housings and boundaries if EMC-rated components at the equipment boundary are not used. The frequency(s) of the leaks will depend upon the geometry of the component and how it is mounted to the housing (e.g., what type and size of EMC seal is used between the component and the housing). The use of components in any digital I&C equipment design should be minimized. No component is completely leak proof. Every component will leak to some degree. Equipment seams and screw penetrations will also leak, but standard EMC design practices exist for essentially preventing them from having significant leaks. Equipment designers need to specify the use of an EMC-rated housing for any digital I&C system regardless of where it is used in the plant.
Another source of immunity leak regarding components that penetrate equipment housings is the normal wear and tear that components must endure. Equipment specifications should also include that EMC-rated components be rated for a specific degree of wear and tare in efforts to preserve immunity. Potentiometers and switches are two components that will undergo wear and tear outside of equipment access panels and ventilation filter panels that should be frequently changed to allow for adequate air flow for cooling equipment.
The Third Layer – The System Cabinet
The system cabinet in a digital I&C system design represents the third layer of EMC protection for immunity. A system cabinet will also help reduce emissions. System cabinets used for digital I&C equipment in advanced nuclear plants should be EMC-rated. Figure 3 illustrates an example of a shielded system cabinet. EMC engineering practices in the area of EMC shielding and gasketing are used when designing these cabinets to ensure that gaps and hardware contain the proper EMC shielding devices.
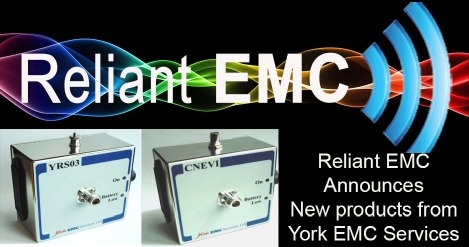
Example of a Shielded System Cabinet
A number of manufacturers of industrial cabinets provide shielded cabinets and shielded seismic cabinets that meet or exceed US Federal Communications Commission (FCC) Class A and B requirements for equipment protection. Utilizing an extrusion based frame system, integrated with beryllium-copper gaskets and shielded ventilation, allows most cabinets to maintain a professional appearance while providing the EMC performance needed in advanced nuclear plants. Designed to perform in the most demanding applications, the bezels are rated to a 6,600-pound static load and corrosion resistance is achieved via durable acrylic coating based on thermo setting, cross linking resins. A wide selection of doors, side panels, top and bottom covers combined with a family of accessory items are also typically available. EMC-grade system cabinets may be tested to requirements outlined in IEC 61000-5-7, Ed. 1, Electromagnetic compatibility (EMC) – Part 5-7: Installation and mitigation guidelines – Degrees of protection provided by enclosures against electromagnetic disturbances (EM code) (2001) or to IEC 61587-3, Ed. 1, Mechanical structures for electronic equipment – Tests for IEC 60917 and IEC 60297 – Part 3: Electromagnetic shielding performance tests for cabinets, racks and subracks, (2006). I&C planning engineers and I&C plant engineers should check to see which EMC test standard that a cabinet is tested before specifying or purchasing the cabinet. Manufacturers should be willing to share cabinet drawings and EMC test results describing the EMC performance of EMC-grade cabinets.
Listed below are some features of shielded cabinets:
- Modular extruded, aluminum frames
- 40 to 100 dB shielding effectiveness (30 MHz to 1,000 MHz)
- Beryllium-copper gasketing applied to all seams and joints (spring and saw-tooth) to insure paint-free electrical contact areas with optional clear chromate
- Shielded mesh ventilation (honeycomb optional)
- Shielded accessories to include: panels, drawers and writing surfaces
- Solid billeted aluminum corners (four way crimped)
- Bezel tested to 6,600-pounds static load (2,000 -pound rated)
- Standard Sizes
- Widths: 19”, 23” and 24”
- Heights: 12U, 16U, 20U, 24U, 30U, 35U, 40U, 44U
- Depths: 24”, 30”, 36”, 42”
- Independent, removable side, top panel, bottom panel and doors
- Integrated cable management, power conditioning and cooling options
- Wide range of standard body and trim color options
Figure 4 illustrates the attenuation of one shielded cabinet versus frequency. One can see that an attenuation level of 48 dB is provided from 30 MHz up to 2 GHz in step with the FCC Class A limit.
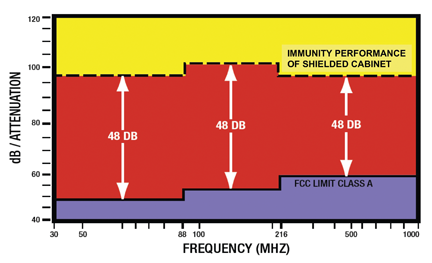
Attenuation of One Shielded Cabinet vs. Frequency
“Leaks” in the Third Layer
Despite all of the good EMC engineering practices that go into designing shielded cabinets, they can certainly leak. As with any other shielding material or system, leaks will form at the joints (e.g., where walls are seamed and at the door seals) and where component penetrate the cabinet wall. Some cabinet manufacturers conduct door-opening-and-door-closing tests to determine when door seals begin to fail due to the opening and closing of EMC-sealed doors. However, leaks can be avoided and minimized if the cabinet is maintained. Once shielded cabinets are installed and mounted, pressure or forces should not be applied to any of their exterior surfaces. Manufacturers will be able to provide any necessary maintenance guidelines to ensure that the EMC immunity is sustained.
Visiting the Size of Exclusion Zones for RF Transceivers
Table 2 calculates the size needed for exclusion zones for various types of portable RF transceivers. For personal communications service (PCS) cellular phones of one- to two watts, a distance of four meters (3.6 meters in Table 2) is required. For four- to eight-watt walkie-talkies, the zone must be over seven (7.2 meters in Table 2) meters.
Alternatives to the Exclusion Zone Strategy
In this section, an alternative strategy to exclusion zones is described.
Enhancing Cabinet and Cable Shielding
Because of the difficulty of establishing and enforcing adequate exclusion zones, EPRI research carried out in 2010 proposed a different guiding logic to achieve the same goal of providing adequate radiated immunity protection to digital I&C equipment from fields generated by portable wireless devices. In this alternate strategy, rather than use distance to lower the emissions from portable wireless devices [3], cabinet and cable shielding is used. The goal here is to provide the required level of radiated EMC immunity for the digital I&C equipment inside the cabinet without having to implement an exclusion zone around the outside of the cabinet.
The digital I&C equipment is still required to have a broadband RF immunity of 10 V/m. A safety margin of 40 dB is recommended, in contrast to the previous 8 dB defined in previous EPRI guidance (see EPRI TR-102323, Rev. 3). The safety margin is increased to provide an increased level of safety, which is achievable because significant levels of shielding effectiveness are obtainable for shielded cabinets at reasonable price levels. A shielding effectiveness of 80 dB is recommended, which is achievable with commercially available shielded enclosures.
The combination, then of a 10 V/m immunity level in the digital I&C equipment, with a 40 dB safety margin and 80 dB of cabinet and cable shielding, results in an RF immunity level greater than 1,000 V/m. This is sufficient to meet even the 600 V/m field strength calculated from an eight-watt device at distance of five (5) centimeters, with margin to spare. Table 3 provides a numerical summary of the above discussion.
Table 4 presents the calculated separation distance at which a portable wireless device will place the current guidance (see EPRI TR-102323, Rev. 3) for an electric field limit of 4 V/m on a digital I&C system inside of an 80 dB shielded enclosure. As can be seen, even the most powerful device must come within 0.7 mm to achieve this level. Certainly, the thickness of the cabinet wall alone will ensure that this distance is always achieved.
Managing the Output Power of RF Transceivers in Advanced Plants
Managing the output power of RF transceivers is obviously a good approach to help reduce the likelihood of developing an EMI problem with digital I&C equipment and systems in advanced nuclear plants. Some necessary actions to reduce the dependence on exclusion zones are discussed below.
Necessary Actions to Reduce Dependence on Exclusion Zones
Immunity Impacts when Opening Cabinet Doors
Doors on system cabinets are routinely opened by I&C engineers for a number of reasons. These reasons include routine equipment inspections and maintenance, calibrations, equipment modifications, and troubleshooting. In many cases as a part of their daily routine, engineers must use portable transceivers to communicate with others in the plant to get the job done. While they do take precautions in today’s existing plants not to key and talk on their portable transceivers while looking closely into cabinets or standing too close to their open doors, the fear of causing the equipment inside the cabinet to trip offline is real.
What happens to the EM immunity level of a digital I&C system when its cabinet doors are open depends a great deal on the amount of EMC shielding protection the cabinet is being called upon to provide. For some systems, with excellent circuit and component sub-system immunity, opening the cabinet doors is of only minor consequence. However, for other systems where almost all of the protection is provided by the cabinets, then opening the doors invalidates that protection and exposes the system to significant risks of interference. Additionally, engineers may have to access some part of the equipment inside the cabinet by removing one or more panels on an equipment housing or by opening a door on a system internal to the system cabinet. When any of the above occurs, electronic circuits and components inside a piece of digital I&C equipment are unprotected and exposed to the radiated environment.
It is necessary to know the EM immunity of digital I&C systems both with the cabinet doors open as well as when they are closed. Some I&C system manufacturers resist testing with the cabinet doors open at times from a fear that it will reveal significant vulnerabilities in their equipment. Others may invite an open-door radiated immunity test simply to learn more about how their equipment will respond without cabinet-level protection. However, a two level strategy of EM immunity is quite feasible.
A higher level of EM immunity can be required with the cabinet doors closed. This will be the normal condition, and so the most likely situation when significant EM fields are imposed on the I&C system. A second and low level of EM immunity could be required with the cabinet doors open. This allows for the open cabinet to participate in the EM protection of the system, but not be the complete solution for both cabinet door conditions. The system with the cabinet doors open should have a level of EM immunity that is adequate to provide protection during plant maintenance activities and other actions that require opening the cabinet doors. This is why it is important to use shielded cables (power and data) inside the cabinet. Because the doors being open will be a less frequent event and almost always have personnel involved with technical training, extra protections can be applied to this condition. This would help to assure that the loss of the protection afforded by the cabinet is compensated for by extra, temporary measures to protect the I&C system.
Transitional Issues
Changing from one EM control system to another is never an easy process. Transitions must be planned and migration paths established to minimize and hopefully eliminate disruption and protect against loss of EM protection. Advanced nuclear plants have the very real advantage that they do not need to support legacy equipment or installations. However, the move to a new EM control system is not without its issues. This section addresses some of those issues and offers guidance on addressing them.
Existing nuclear plants must support installed systems and legacy equipment. When engaging in a digital I&C upgrade, migration paths must be planned which continue to support existing installations. These plans must assure that existing systems continue to give adequate levels of EM protection, while also allowing a change to new equipment and system, with better levels of EM immunity.
The need for migration paths is not eliminated with advanced nuclear plants. The control systems used by current plants have a history and documented performance. Albeit, in some cases that history shows there is a need for improved performance as EMI and other problems have occurred and are documented. Moving to a different EMI control system carries the risk that the new systems will perform as intended.
If increased levels of EM immunity are required, there is the risk that the standards and tests that are used to measure that immunity may be flawed, allowing equipment to pass which does not provide the required level of immunity. New standards have the risk that they may not adequately evaluate the EM immunity of equipment.
A different but related risk is that EMC test engineers and laboratories may not understand and correctly test to a new standard. There is a learning curve and mistakes are to be expected. Further, many EMC labs that perform this testing are not accredited to do so. Test results from non-accredited test labs are particularly at risk of having deficiencies. New standards must be implemented with adequate training for test engineers and time for laboratory accreditation bodies and quality managers to incorporate the new requirements into their testing and documentation processes. It is always the nuances and special cases that prove particularly problematic. Carefully implemented transition plans are necessary to assure that the required levels of protection are safeguards throughout the process.
A complete transition plan is necessary with appropriate quality checks and feedback mechanisms to assure that an improved EM control system is implemented in a way that assures the high levels of protection as safeguarded throughout the entire process and into the future.
Conclusion
This article, Part 2 of 2 on the topic of exclusion zones and their strategies in nuclear power plants, described the elements of the current exclusion zone strategy used in existing plants and the logic behind it. Unfolding the layers of immunity included in an I&C equipment and system applications illustrated the limitations of the current exclusion zone guidance. Unfolding the layers of immunity also showed how the this approach can be used to understand how each layer provides a necessary part of whole-system immunity including that offered by EMC-grade shielded cabinets. Typical RF power levels from four types of intentional radiators were used to demonstrate the distances needed between portable transceivers and the I&C equipment enclosed in a non-EMC-grade system cabinets. Conversely, this same process was repeated while including a lower-risk safety margin of 40 dB and an 80 dB level of protection offered by shielded cables and a shielded cabinet. The results were exclusion zone sizes on the order of tens of millimeters instead of tens of meters. Millimeter-sized exclusion zones around a cabinet will be covered by the thickness of the cabinet walls. Planners for new advanced nuclear plants and their I&C engineers should include the use of EMC-grade shielded cabinets in the design of the digital I&C systems—a requirement that is paramount to avoiding EMI problems in advanced plants. Modification engineers working on digital I&C upgrades in existing plants should also apply this new logical guidance when designing a new digital I&C system to replace existing analog I&C systems.
Notes
- Guidelines for Electromagnetic Interference Testing in Power Plants: Revision 1 to TR-102323-R1, EPRI, Palo Alto, CA, January 1997.
Guidelines for Electromagnetic Interference Testing of Power Plant Equipment: Revision 2 to TR-102323, EPRI, Palo Alto, CA, November 2000, 1000603.
Guidelines for Electromagnetic Interference Testing of Power Plant Equipment: Revision 3 to TR-102323, EPRI, Palo Alto, CA, and the U.S. Department of Energy, Washington, D.C., 2004, 1003697. - U.S. Nuclear Regulatory Commission, Guidelines for Evaluating Electromagnetic and Radio-Frequency Interference in Safety-Related Instrumentation and Control Systems. NUREG/1.180, Rev. 1, October 2003.
- Portable wireless devices are emphasized because they are inherently harder to control because their location is not fixed. It is assumed that adequate protection to portable devices will consequentially give protection to fixed wireless transmitters. There are potential exceptions to this assumption, such as if a special purpose, unusually high powered transmitter is installed near I&C systems. It is assumed that plant EMC engineers will be alert for such possibilities and take appropriate steps if exceptional situations present themselves.
Bibliography
Guidelines for Electromagnetic Interference Testing in Power Plants, EPRI TR-102323, Rev 1, Electric Power Research Institute, Palo Alto, CA, 1996.
Guidelines for Electromagnetic Interference Testing in Power Plants, Revision 2 to EPRI TR-102323, TR-1000603, Electric Power Research Institute, Palo Alto, CA, 2000.
Guidelines for Electromagnetic Interference Testing in Power Plants, Revision 3 to EPRI TR-102323, TR-1003697, Electric Power Research Institute, Palo Alto, CA, 2004.
Guidelines for Evaluating Electromagnetic and Radio-Frequency Interference in Safety-Related Instrumentation and Control Systems, Regulatory Guide (R.G.) 1.180, U. S. Nuclear Regulatory Commission, 1996.
Keebler, Philip F., “Eliminating the Need for Exclusion Zones in Nuclear Power Plants.” IN Compliance, June 2011.
Keebler, Philip F. and Stephen Berger, Technical Guidance for Achieving Higher Levels of Electromagnetic Compatibility for Advanced Nuclear Power Plants: Addressing the EMC Challenges for Reliable Plant Operation, EPRI, Palo Alto, CA, 2010, 1021092.
![]() |
Philip Keebler is a Senior Research Engineer in the Power Delivery & Utilization Sector at EPRI with one focal area on electromagnetic compatibility (EMC). Philip received his M.S. and B.S. in Electrical Engineering from the University of Tennessee. Philip is active in many professional organizations, including but not limited to IEEE, Illuminating Engineering Society of North America (IESNA), and the Association for the Advancement of Medical Instrumentation (AAMI), and is Chair of the TC-4: EMI Control within the IEEE EMC Society. Mr. Keebler is also currently working on new EMC standards for the nuclear power plant industry. |