High-Altitude Electromagnetic Pulse Protection for Critical Electronic Systems
The modern world as we know it is ruled by technology. Every aspect of life today is encompassed by some sort of microprocessor-based electronic device intended to simplify tasks, reduce processing times, increase efficiency, and improve accuracy. Electronics are used to communicate with loved ones, manage finances, fly aircraft, autonomously drive us to work, and even save lives. As we achieve further advances in technology, electronics will continue to lead the way in adapting these technologies into new critical systems and processes.
The benefits that electronics have provided us are undeniable. However, there is a significant drawback as the implementation of sensitive electronic circuitry continues to increase our vulnerability to the effects of electromagnetic threats such as an EMP attack. An electromagnetic pulse (EMP) is defined as a high amplitude, short duration, broadband pulse of electromagnetic energy that can have devastating effects on unprotected electronic equipment and systems. EMPs are historically known as the electromagnetic effects following a nuclear blast occurring at high altitudes.
The Science Behind EMP
Initial research of the electromagnetic effects was documented by the U.S. in 1958 during a series of high-altitude atmospheric tests. The most noted was during the detonation of the nuclear payload named “Starfish Prime” over the Pacific Ocean over 800 miles away from Hawaii. It produced a severe electromagnetic pulse that traveled distances much further than the shock wave and blast effects that were reported to have disrupted radio stations, damaged electrical equipment, and even blew out streetlights along the shoreline of Hawaii.
A high-altitude nuclear electromagnetic pulse (HNEMP) develops from the detonation of a nuclear bomb at very high altitudes above the surface of Earth. The blast releases a burst of gamma rays that travel at the speed of light in all directions from the detonation. When these gamma rays enter Earth’s atmosphere, they collide with oxygen atoms, causing a scientific phenomenon known as “Compton scattering” to occur. When a high energy light photon collides at a molecular level with one of the atom’s electrons, it transfers some of its energy and changes its trajectory. This collision also sends the negatively charged electron on an accelerating trajectory away from its positively charged nucleus.
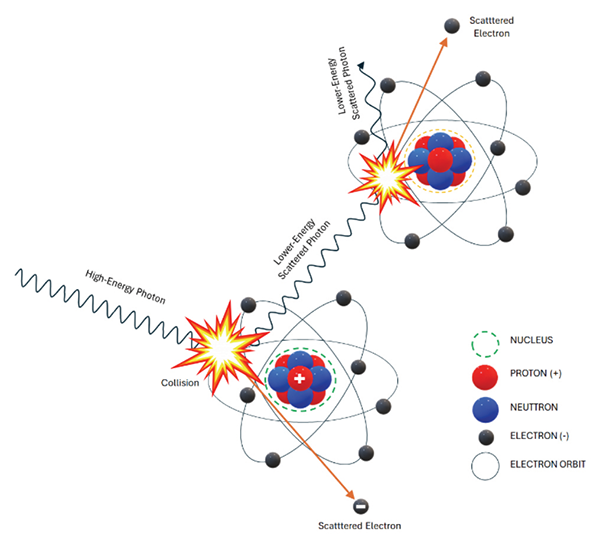
Commonly known as a “Compton electron,” this electron spirals along the Earth’s magnetic field lines, resulting in rapidly varying electric and magnetic fields, which produces a large, fast-rising asymmetric electric field pulse. Additionally, the photon will continue to collide with other nearby air molecules in its trajectory path until it is depleted of energy. Secondary electromagnetic pulses caused by the interaction of the initial pulse with the Earth’s surface and the ionosphere may occur as well.
The exposure radius of a high-altitude EMP, commonly known as the “disposition region,” is determined by three main elements:
- The height of the blast;
- The size of the blast; and
- The type of explosion.
In general terms, the disposition region increases with the altitude of the detonation. Altitude also plays a role in the asymmetry of the pulse waveform as well. The size and type of blast will determine the magnitude of the EMP. Theoretically, the size of the EMP disposition region is only limited by the curvature (horizon) of the planet.
It has been theorized that a 100-megaton nuclear payload detonated at a height of approximately 300 miles over the state of Kansas (central United States) would result in an EMP disposition region large enough to envelope the entire country. A HNEMP from this height would extend to the visual horizon of the planet as seen from the burst point perspective (see Figures 2 and 3).
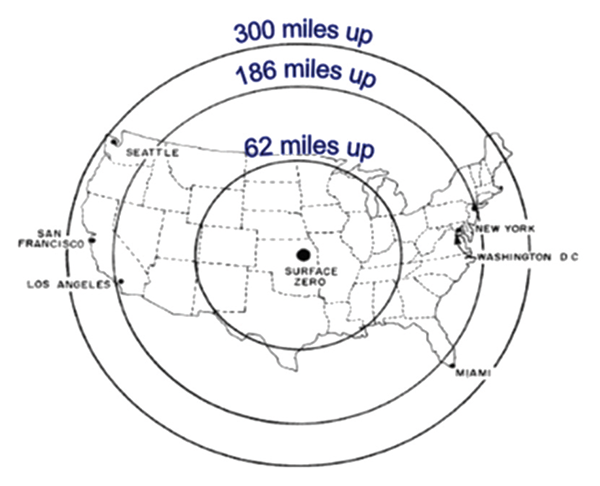
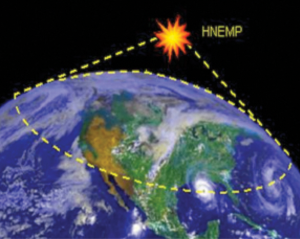
About HNEMP
A HNEMP is a complex multi-pulse waveform containing a broad spectrum of electromagnetic radiation spanning a range from a few Hz to several GHz.
The pulse is defined in terms of three components:
- E1 Early Time Pulse: Also defined as the prompt gamma signal, E1 early time pulse is characterized by its fast rise time (2-10 nsec) and peak values between 50 kV/m – 100 kV/m. The E1 early time component is used as an EMP evaluation tool as its fast rise time exceeds the reaction time for commonly integrated lightning protection devices. The E1 pulse magnitude can temporarily disrupt or permanently damage the operation of electronic devices due to high induced voltages and currents. The E1 pulse frequency spectrum couples to RF antennas and receiver ports, equipment enclosures (through apertures), and short and long cabling and power lines.
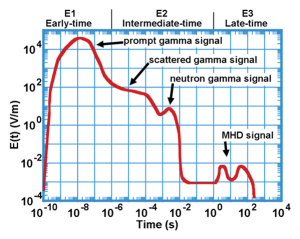
- E2a Intermediate Time Pulse: Related to the scattered gamma signaling effects, E2 pulse operates around 1/1000 µsec, with peak values in 100 V/m range. Characteristics are similar to low-level indirect lightning strikes, and common lightning surge protectors will help mitigate E2 EMP pulses coupled to power lines and antenna ports, including long power lines, vertical antenna towers, and aircraft with trailing wire antennas.
- E2b Intermediate Pulse: Also referred to as the neutron gamma signal, E2b pulse durations are long and slow, around 1 second, with peak values in the 10 V/m range. Effective coupling to long overhead and buried power lines and to extended VLF and LF antennas can mitigate the effect. However, dominant frequencies overlap AC power and audio spectrums, making filtering difficult.
- E3 Late Time: Referred to as the Magnetohydrodynamic (MHD) signal, the E3 signal has a very long duration, ranging from 50 minutes to several hours. E3 signal amplitude is quite low, around 100 mV/m. The E3 signal characteristics are similar to geomagnetic solar storms and flares.
Due to the long duration of the E3 signal, disruptive geomagnetically induced currents (GIC) can occur in power transmission lines that can affect the normal operation of long electrical conductor systems such as electric transmission grids, buried pipelines, oil and gas pipelines, non‑fiber optic undersea communication cables, non-fiber optic telephone and telegraph networks, and railways. The associated electric field (measured in V/km) acts as a voltage source across conducting networks. GIC are often described as being quasi-direct current (DC), although the variation frequency of GIC is governed by the time variation of the electric field.
Weapons Leveraging EMP Technologies
While a high-altitude nuclear electromagnetic pulse attack may seem unlikely, at least in the near term, non-nuclear weaponized EMP technologies have been progressively developed worldwide for decades. These technologies are classified as direct energy weapons (DEW) and are currently being used today by our U.S. armed forces and government agencies worldwide.
DEWs are designed to emulate the HNEMP E1 pulse characteristics and direct this at the target, much like that of a conventional EMP, at close range. Some DEWs have been shown to be capable of specialized tuning fork graduated effects on specific electronics types ranging from operational disruption, permanent hardware damage, and complete destruction of systems.
A prime example of this technology is the “anti-drone rifle” or “anti-drone gun,” a battery-powered electromagnetic pulse weapon that is shoulder-fired and intended for disabling flying drone targets (see Figure 5). The device emits separate electromagnetic pulses to suppress navigation and transmission channels used to operate the drone and terminate the drone’s contact with its operator, ultimately resulting in the out-of-control drone crashing.
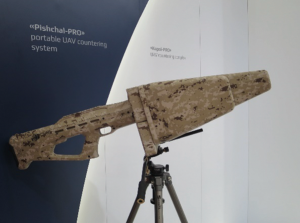
A similar example of this is the Russian “Stupor,” a shoulder-fired EMP gun reported to have a range of two kilometers, covering a 20-degree sector. This device also suppresses the drone’s cameras. The Stupor is reported to have been used by Russian forces during the Russian military intervention in the Syrian civil war in 2011.
Yet another example of non-nuclear EMP technology is the flux compression generator (FCG). The FCG was first demonstrated by Clarence Fowler at Los Alamos National Laboratories (LANL) in the late fifties. This technology injects a high-energy pulse into a large conductive coil. At the point of peak pulse current, a small explosive charge is deployed, which rapidly compresses the coil to one end of the generator, developing a massive amount of electromagnetic energy (see Figure 6). The first designs were several feet in length but, through technological advances, they are now reported to be roughly the size of a beer can.
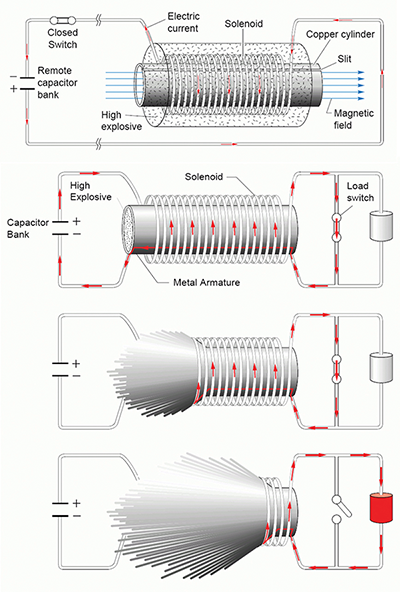
The U.S. Navy used an FCG pulse weapon (see Figure 7) during the opening hours of the Persian Gulf War to effectively destroy vast amounts of Iraqi electronics, power, and telecommunications system infrastructure quickly and efficiently. The deployment of EMP weaponry instantly caused what is known as the “Fog of War” (a complete loss of communications between troops and command posts), which devastated the Iraqi forces, essentially ending the war before it began.
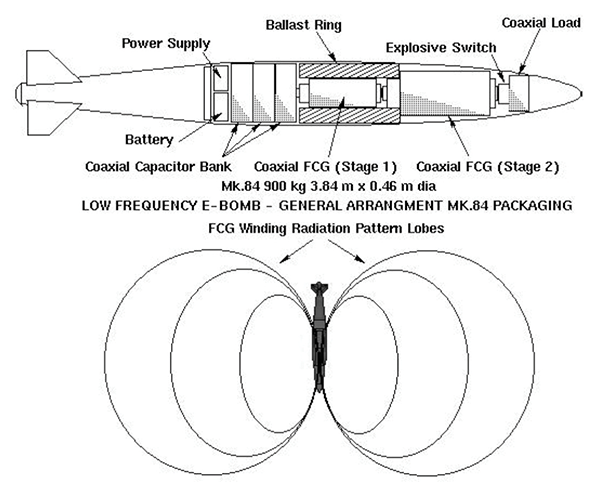
Testing for EMP Resilience: MIL-STD-461G, RS105 Test Method
With the creation of non-nuclear direct energy weapons and the increased use of devices on the battlefield and in civilian non-combat environments, the need to protect electronic equipment is at an all-time high. There are a number of well-established standards and design guidelines available for hardening equipment and systems against the effects of a weaponized electromagnetic pulse attack.
MIL-STD-461G provides test methodology and screening levels for determining a device’s immunity to EMP from a radiated and conducted standpoint. The coupling modes onto the equipment enclosure and its interconnecting cabling can be complex and, therefore, are evaluated separately.
The RS105 test method specified in MIL-STD-461G addresses the risk of radiated exposure to an EMP event. RS105 testing is generally applicable for equipment installed in exposed and partially exposed environments. The U.S. Department of Defense (DoD) requires an electromagnetic environmental effects (E3) assessment for nearly every platform, from surface ships, submarines, and aircraft to space systems and ground applications, including fixed and mobile mission critical C4i facilities.
The RS105 electromagnetic pulse characteristics consist of a fast rise time, short pulse duration, and high amplitude, which resemble the E1 early-time characteristics of a HNEMP. Peak field strengths of 50 kV/m -0 + 6dB are specified for equipment that may be exposed or partially exposed to an EMP once deployed. However, tailoring the peak field levels is often required for partially exposed installations due to the attenuated effects provided by enclosures, such as the deckhouse structure or hangar doors.
For example, equipment installed on a ship near a deckhouse aperture is required to meet the full EMP threat environment reduced by the aperture’s partial electromagnetic shielding effectiveness or 40 dB of shielding provided by the deckhouse structure, whichever is less. In other cases, the EMP field levels may be increased to determine the resiliency threshold of a particular device or to assess the linear effectiveness of its EM shield.
Equipment-level EMP exposure testing to RS105 testing is performed with a transmission line connected to a transient pulse generator (see Figure 8). The EMP generator, which basically consists of a high voltage DC source, high voltage capacitor bank, and a switch, is connected to one end of the transmission line and referenced to ground. The far end of the transmission line is then securely bonded to the ground plane. This ground connection provides a return path, allowing current flow and the generation of electromagnetic fields to develop within the transmission loop.
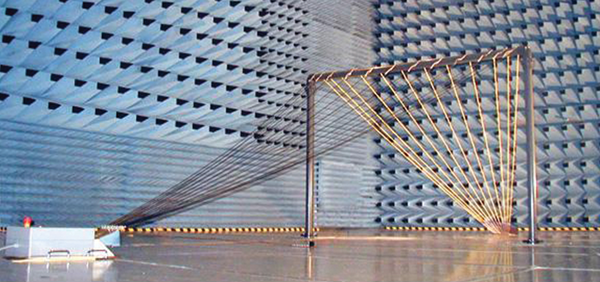
The field developed between the transmission line and the ground plane consists of a large differential voltage and current field. MIL-STD-461G also requires that the EMP field is uniformly distributed over the test article (see Figure 9). Therefore, the field is verified at 5 points vertically based on the maximum dimensions of the equipment under test (EUT). The results taken at each point must be within -0 to 6dB tolerance. In terms of voltage, the uniform field level between all measured points shall be between 50,000 and 100,000 V/m.
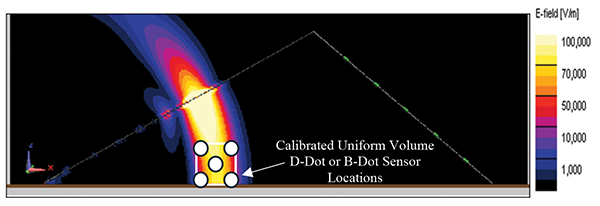
The purpose of RS105 testing is not to damage the equipment but to determine its operational threshold level to the electromagnetic pulse. Here, testing is performed at a very low pulse level (500 V/m or 10% of the maximum peak field level), then gradually increased until either susceptibility is observed, or the specified peak field level is reached.
It is important to note that RS105 testing is intended to evaluate the effects of a radiated EMP only. Therefore, the test setup requires that all metallic interconnecting cabling, including power input lines, are routed in shielded conduit and/or underneath the ground plane and filtered as necessary to minimize EMP coupling effects.
Testing for EMP Resilience: MIL-STD-461G, CS 116 Test Method
The MIL-STD-461G CS116 test method is then used to evaluate the coupling effects of EMP on metallic interconnecting lines. The intent of this test is to ensure the equipment’s ability to withstand conducted damped sinusoidal transients excited by platform switching operations, indirect effects of lightning, and EMP. The minimum set of test frequencies includes 10 kHz, 100 kHz, 1 MHz, 10 MHz, 30 MHz, and 100 MHz, which broadly covers the EMP critical frequency spectrum. In accordance with MIL-STD‑461G, CS116 testing is applicable for all installation platforms and procurement agencies, with limited applicability for submarines.
Similar to RS105, CS116 testing is used not to damage the equipment but to determine its operational threshold to the coupling effects of an electromagnetic transient pulse. This is performed by starting at 10% of the peak field level and gradually increasing the field until susceptibility is determined or the specified peak field level is reached.
One important aspect to note about this testing method is that the transient signals are inductively coupled to each line separately. In an actual fielded exposure scenario, all interconnecting lines are likely to be exposed simultaneously. Additionally, the amount of voltage and current induced onto each line is dependent on its common mode impedance. Higher impedance lines will allow for greater voltages to be achieved at lower currents, whereas low impedance lines, such as shielded cabling, will achieve greater currents at lower voltages.
To avoid excessive over-testing, pre-calibration of the injected currents into a 100-ohm loop impedance is performed, and the currents induced onto each line are monitored. As mentioned, test levels are gradually increased until equipment susceptibility is detected and the current limit is achieved, or the generator setting determined during the 100-ohm calibration is reached.
Testing for EMP Resilience: MIL‑STD-188-125
MIL-STD-188-125-1 & -2 outline the unclassified high altitude electromagnetic pulse (HEMP) protection control requirements for fixed and mobile ground-based command, control, communications, computer, and intelligence (C⁴I) systems and facilities. EMP protection performance in accordance with this standard is assessed in multiple phases:
- Shielding effectiveness (SE) testing
- Pulsed current injection (PCI) testing
- Continuous wave (CW) immersion testing
- Threat level illumination EMP testing
Shielding Effectiveness Testing
Shielding effectiveness (SE) testing has long been used to evaluate the performance of an electromagnetic shielded boundary used to suppress radiated electromagnetic signals entering or leaving the shield’s inner boundary. There are numerous industry standards and SE test methods, such as those found in IEEE-STD-299 that can be used to assess the performance of a large, shielded equipment enclosure intended specifically for protection from an external EMP event.
From a basic sense, transmitting and receiving antennas are first centered on each other at a set distance. The antenna type and frequency band being tested are normally matched. The instrumentation is then stepped through the measurement frequencies while forward power data and transmitted signal emissions readings are recorded. This establishes a baseline to determine the SE value (see Figure 10).
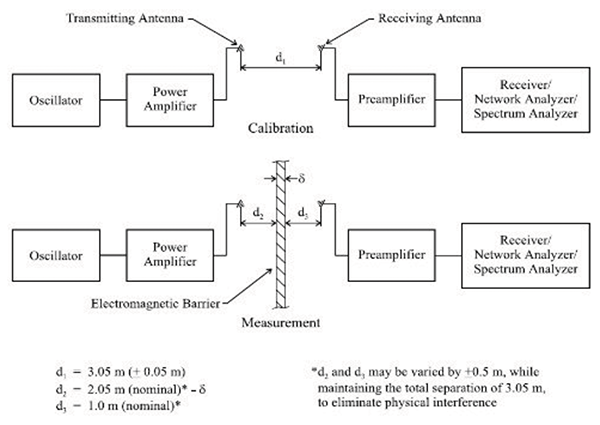
Depending on the location where testing is being performed, the baseline measurement may need to be performed outdoors or in an uncontrolled electromagnetic environment. In these cases, it becomes critical to account for sufficient dynamic range when establishing the baseline reading, as it is likely that the ambient electromagnetic environment will interfere with these readings.
A general rule of thumb is to ensure that the dynamic range is at least 6 dB greater than the anticipated SE value over the test frequency range. It is common to level the baseline value across the test frequency spectrum by adjusting the transmitter’s forward power level. This approach may simplify the SE calculation process when in the presence of a noisy ambient background environment.
Once the baseline is established, the antennas are positioned on either side of the shielded boundary. The shielding effectiveness measurement axis (the imaginary line between the transmitting antenna and receiving antenna locations) for each test area shall be normal to the shield surface and shall pass through the geometric center of the area. Additionally, the same spacing between the two antennas used during the baseline measurement must be observed during the SE measurement, as this will impact the measured results.
Once completed, the baseline power level is replayed, and the attenuated signal level is received. The SE value is then calculated by simply subtracting the attenuated signal measurement data from the baseline data (see Figure 11).
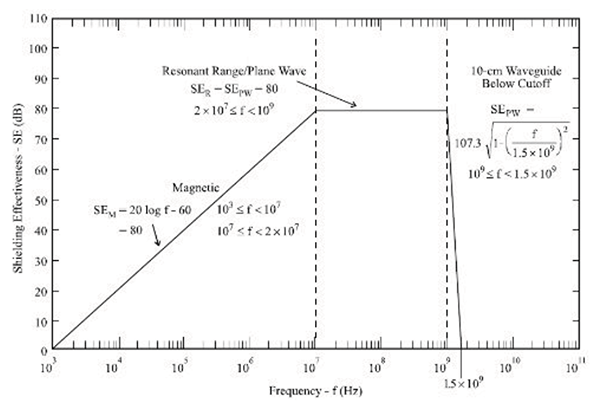
This process is repeated multiple times along the enclosure’s entire surface area (including the floor when both sides of the shield are accessible). MIL‑STD-188-125 requires that the minimum number of positions should be divided into numbered plane areas no greater than 3.05 m × 3.05 m (10 ft × 10 ft). However, specific mechanical design elements, including seams and aperture features that could constitute a weakness of the electromagnetic boundary, must be tested.
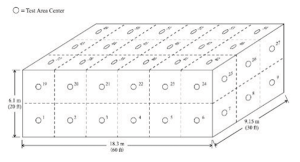
It should be noted that, unlike a radiated emissions control specification, SE performance should either meet or exceed the limit requirements versus having some margin below the limit specification. The higher the shield’s SE performance, the greater its suppression of an external EMP field.
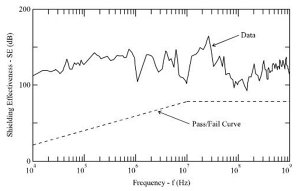
SE data is also incredibly useful during EMI/E3 system risk assessments during the early stages of development. Assuming SE data can be applied linearly to an external field response, the internal environment of the shielded boundary can be determined based on an external electromagnetic environment of any level within the measurement frequency range.
Pulsed Current Injection (PCI) Testing
Pulsed current injection (PCI) testing assesses all electrical point-of-entry (POE) protective devices installed on cabling interfacing with low-risk HEMP ground-based facilities. It is used to demonstrate that electrical point of entry (POE) protective devices provide adequate transient suppression/ attenuation of externally coupled EMP currents in accordance with MIL-STD-188-125. Much like test method CS116 for equipment-level testing, compliance with this test demonstrates that mission-critical systems (MCS) are not damaged or upset by residual internal transient stresses caused by an external EMP event.
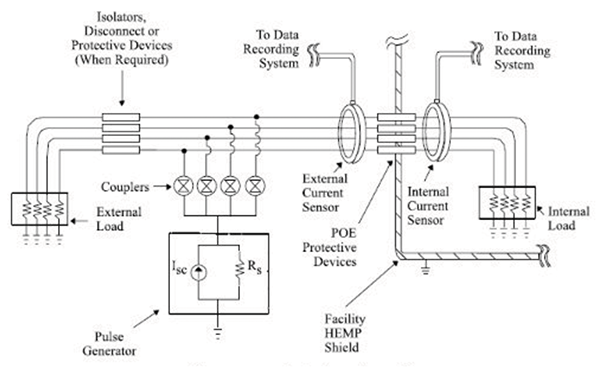
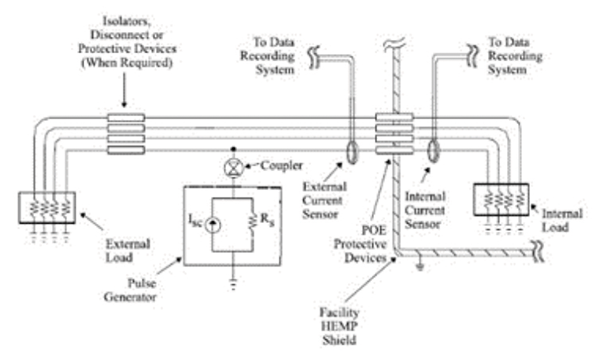
The PCI tests are conducted by injecting threat-relatable transients to conductors at injection points outside the electromagnetic barrier. This is performed on each penetrating conductor and cable, radio frequency (RF) antenna shield, and conduit shield. Unlike test method CS116 testing, simultaneous injection of all electrical POE protective devices is preferred when practical, as this best represents the effects of an actual external EMP event. Injections are also performed in both common mode (all penetrating conductors of a cable simultaneously driven with respect to ground), and individual wire-to-ground configurations are required. Residual internal responses are then measured, and the operation of the system is monitored during the test for indications of mission-aborting damage or upsets.
Conclusion
The development of electromagnetic pulses is very complex, and the effects it can have on electronic equipment and systems can be devastating. This poses an operational threat to mission-critical processes and infrastructures worldwide. It is incredibly important to establish and implement EMP-hardened design features to increase system-level resiliency against potential EMP attacks now.
To successfully navigate the design, assessment, and validation processes to ensure EMP resilience, we strongly recommend working with an experienced third-party testing organization with knowledgeable subject matter professionals expert in EMP-specific issues. Doing so will help to ensure the resilience of your technology against EMP threats and enable you to bring high-quality technologies to market more efficiently and cost-effectively.