In this month’s EMC Concepts Explained, we explore the conducted immunity test known as a Bulk Current Injection (BCI) test, according to ISO 11452-4 specification [1]. This immunity test is carried out by inducing disturbance signals into the wiring harness by means of a current injection probe, as shown in Figure 1.
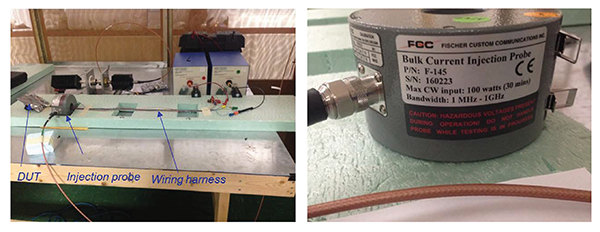
The injection probe is a current transformer through which the wiring harness (which forms the secondary winding) of the device under test (DUT) is passed. Immunity tests are carried out by varying the test severity level and frequency (1 MHz – 400 MHz) of the induced disturbance. The disturbance is an amplitude modulated (AM), narrowband, continuous wave (CW) carrier signal.
The ISO11452-4 standard specifies two BCI test methods: the substitution (open-loop), and current monitoring (closed-loop) probe methods. Both test methods consist of two phases:
- Calibration (using a calibration fixture)
- Testing (using the DUT).
Calibration Fixture and Equipment
The calibration fixture, used in both methods, is shown in Figure 2.
During the calibration procedure (same for both methods), the injection probe is mounted inside the calibration fixture. The calibration fixture is terminated by a 50 Ω load at one terminal and connected to the spectrum analyzer through an attenuator (to protect the measuring equipment) at the other terminal, as shown in Figure 3.
The 50 Ω load and a 20 dB attenuator are shown in Figure 4.
The calibration procedure (and the BCI test) is performed in a shielded enclosure, using several additional pieces of equipment shown in Figure 5.
The block diagram of the test equipment configuration (outside the shielding enclosure) is shown in Figure 6.
During the calibration procedure we need to measure forward power supplied to the injection probe (mounted in the calibration fixture) and the power reflected from it (reverse power). This is accomplished with the help of a directional coupler shown in Figure 7.
The coupler is rated for specific power handling, frequency range, and attenuation. Ports J3 and J4 sample the power and attenuate it before the power sensors can read it. The coupler shown is rated for 100 W, 0.1 – 1000 MHz, 40 dB.
Calibration Procedure
The calibration procedure (same for both methods) is performed with an unmodulated sinusoidal RF signal. During the calibration procedure net power (forward power minus reverse power) is injected into the current probe creating the required current level in the calibration fixture over the required frequency range (1 – 400 MHz). The level of forward power used during the calibration is later used during the actual testing. BCI test severity levels (required current levels) are specified in Table 1.
These limits are displayed in Figure 8.
Maximum frequency step sizes during the calibration should be not greater than the maximum frequency step sizes used in testing, and shown in Table 2.
Frequency band (MHz) | 1 < f ≤ 10 | 10 < f ≤ 200 | 200 < f ≤ 400 |
Linear step (MHz) | 1 | 5 | 10 |
Table 2: Maximum frequency step sizes
AM Modulated Signal
During the testing (in both methods) the AM modulated signal is used with the carrier frequency, fC, varied from 1 to 400 MHz. Let’s briefly discuss the modulation process. The unmodulated signal is a sinusoid shown in Figure 9a.
The modulating signal, shown in Figure 9b, is also a sinusoid of an angular frequency θ, corresponding to the linear (cyclic) frequency of 1 kHz. The amplitude of this sinusoid is controlled by the modulation index, m, where,
The resulting AM-modulated signal is shown in Figure 9c. The peak amplitude of this signal is larger than the amplitude of the original unmodulated signal (since we are adding two sinusoids).
For peak test level conservation, the peak amplitudes of the unmodulated and AM-modulated signals are made equal. That is, the peak amplitude, AC, of the unmodulated signal is reduced to a new level of A′C, so when the modulating signal, m(t), is added to the reduced-amplitude unmodulated signal, the resulting AM-modulating signal has the same peak amplitude as the original unmodulated signal. This is shown in Figure 10.
The peak conservation is achieved with m = 0.8. This AM modulation used in conducted immunity testing is often referred as AM 1 kHz, 80%.
The reduction in the peak amplitude of the original unmodulated signal can be also described in terms of the mean power. Let’s denote the mean power of the original unmodulated signal as PCW, and the mean power of the amplitude-reduced unmodulated signal as P′C. Then [1],
For the above relation becomes
or
Thus, to adjust the amplitude of the carrier wave prior to modulation, the power is decreased by 5.1 dB and then AM modulating signal is added.
Test Procedure and Setup – Substitution Method
The test configuration for the substitution method is shown in Figure 11 and Figure 12, respectively.
Note that the injection probe is placed at three different locations: 150, 450, and 750 mm from the DUT. For a DUT remotely grounded (vehicle power return line longer than 200 mm) two Line Impedance Stabilization Networks (LISNs) are used, (see [2] for LISN description and operation).
The test is conducted by injecting the forward power (recorded during the calibration procedure) into the current probe clamped over the harness connected to the DUT. The forward power is incrementally increased until the level used in calibration (that produced the required current) is reached. Note that the calibrated level of the forward power corresponded to the load of 50 Ω. The load impedance during the testing varies and could be smaller or larger than 50Ω. Thus, the forward power used during the testing may induce larger or smaller currents in the harness than the forward power induced during the calibration.
Test Procedure and Setup – Closed-Loop Method with Power Limitation
The test configuration for the closed-loop method with power limitation is shown in Figure 13 and Figure 14, respectively.
Note that this method uses two current probes one for injection (same as in the substitution method) and the other for current measurements (see [3] for current probe measurements). Such a probe is shown in Figure 15. The injection probe is placed 900 mm from the DUT while the measurement probe is placed 50 mm from the DUT.
During testing power level recorded during the calibration is applied to the injection probe. The forward power is incrementally increased until the level used in calibration (that produced the required current in the 50Ω fixture) is reached. Current level in the wiring harness is measured using the measurement probe. If this measured current is below the level specified in Table 1, the power to the injection probe is increased until the specified current level is achieved or the power limit is reached. The power limit is specified by
where k is a factor equal to 4 unless otherwise specified in the test plan.
Conducted Immunity Test Results
Figure 16 shows the passing test result for a DUT which was tested to the severity level IV, using the substitution method.
The current curve riding on the Level IV curve shows that there were no anomalies when the current reached the severity level IV. To be more precise, there were no anomalies when the forward power applied during testing reached the level used during the calibration. The actual current in the harness during the testing is not known (unless an optional current measuring probe is used).
Figure 17 shows the test result with anomalies for a DUT which was tested to the severity level IV, using the substitution method.
The dip in the current curve indicates an anomaly. When anomaly occurs at a given frequency, the power level is incrementally reduced until the anomaly is no longer present. Then the power level is incrementally increased until the anomaly occurs. At that time the anomaly is marked on the plot (orange dots in Figure 17). Subsequently the frequency is stepped up to the next value and the power is incrementally increased until the required power level is reached or another anomaly is observed.
Figure 18 shows the test result when a DUT was tested using the closed-loop method with power limitation (note: the test limits in this plot do not correspond to the ISO 11452-4 specification).
Notice that up to a frequency of 10 MHz the induced current did not reach the required level, even though the maximum power (equal to 4 times the calibration power was applied).
Functional Status Classification
Anomalies are categorized as belonging to one of several classes. ISO 11452-1, [4], defines theses classes as follows.
- Class A – all functions of a DUT perform as designed during and after exposure to a disturbance.
- Class B – all function of a DUT perform as designed during exposure; however, one or more of them can go beyond a specified tolerance.
- All functions return automatically to within normal limits after exposure is removed (memory functions belong Class A).
- Class C – one or more functions of a DUT do not perform as designed during exposure but return automatically to normal operation after exposure is removed.
- Class D – one or more functions of a DUT do not perform as designed during exposure and do not return to the normal operation until exposure is removed and the DUT is reset by a simple operator action.
- Class E – one or more functions of a DUT do not perform as designed during and after exposure and cannot be returned to proper operation without repairing or replacing the DUT.
Based on the DUT intended function, with respect to the conducted immunity, the DUT is usually also classified (by the manufacturer) as belonging to one of the test groups. These test groups reflect the severity of the DUT malfunction on the safety or functionality of other systems.
During the immunity testing, each test group must meet a set of minimum requirements defined by the functional status classification above.
References
- ISO 11452-4:2011(E) – “Road vehicles – Component test methods for electrical disturbances from narrowband radiated electromagnetic
energy – Part 4: Harness excitation methods”. - Adamczyk, B., Teune, J., “Topology and Characterization of a DC Line Impedance Stabilization Network”, In Compliance magazine, July 2017.
- Adamczyk, B. “Current Probe Measurements in EMC Testing”, In Compliance magazine, September 2017.
- ISO 11452-1:2005/Amd.1:2008(E) – “Road vehicles – Component test methods for electrical disturbances from narrowband radiated electromagnetic energy – Part 1: General principles and terminology.”