Underwriters Laboratories did basic research in the field of safety and published the results of that research in a series of “Bulletins of Research.” At least 58 bulletins were published relating to fire, explosion, and electric shock. One of those Bulletins, “Electric Shock as it Pertains to the Electric Fence,” is a classic document in the field of product safety. The research was performed from 1936 to 1939 by Baron Whitaker, an Assistant Electrical Engineer at UL. Whitaker ultimately ascended to the presidency of UL.
Whitaker’s research still stands today. While similar research has been done in support of modern IEC publications, such research is usually published only in IEC committee papers and is usually highly focused towards the specific standard or report the IEC is attempting to write.
This UL Bulletin of Research on the electric fence contains much information that applies to much more than just the electric fence. This is why it is a classic work.
INTRODUCTION
Whitaker introduces his research by describing the electric fence: “One of the most recent and novel applications of electricity in the rural areas today is the electric fence. Physically, the electric fence differs from the conventional type of barbed-wire or woven-wire fence in that it is of simpler construction (usually having one wire) and does not require the mechanical strength or stability of the older types. Functionally, it is different in that it controls animals by means of fear rather than by strength or by causing pain. The electric fence is composed of two distinct parts, namely, the fence wire, and the electric controller which supplies the electrical energy to the fence wire.”
PURPOSE AND CONDITIONS
Whitaker studied electric fence accident reports involving both electric shock injury and electric-shock-caused death. Based on his study, he bounded his research by stating that the electric fence should be safe for a two-year-old child, “…barefooted, standing in a pool of water or mud, and falling across or grasping the wire with two wet or sweaty hands, the wire, so far as the child is aware, being an ordinary non-electrified fence wire.”
(Anecdotally, a colleague, whose home is a farm and uses electric fences, reported that these were the conditions under which his wife rescued his daughter!)
Under these conditions, Whitaker wanted to determine the maximum value of current, for both ac and dc, the frequency, and the duration that “can be considered as not being hazardous to human life.”
Whitaker undertook to determine values for:
- Body electrical resistance
- Safe open-circuit voltage
- Effects of dc, interrupted dc, ac, and frequency of ac
- The maximum current and duration which will not cause bodily injury
- The minimum off time
THE NATURE OF ELECTRIC SHOCK
Whitaker researched the various causes of death from electric energy. He found five different causes:
- Paralysis of the respiratory muscles, producing death from asphyxia
- Hemorrhage, produced by increasing blood pressure during the passage of electric current
- Heart failure, produced by ventricular fibrillation
- Respiratory failure, produced by nervous inhibitions or actual damage to the nervous system
- Skin and flesh burns, with resultant complications
Whitaker’s research was oriented towards prevention of one or more of these injuries. He was not dealing with prevention of sensation or prevention of reflexive action as we do in today’s products. Furthermore, electric fence manufacturers claimed that an effective fence should provide sufficient current to cause muscle contraction, and that the “off” period should be as short as possible.
BODY ELECTRICAL RESISTANCE
Whitaker starts his consideration of body resistance with the statement, “Necessary to the establishment of the safe operating characteristics of electric fence controllers is a consideration of the human body as a conductor of electricity.”
Whitaker initiated a series of tests at UL to measure body resistance. Whitaker asserts that “the outer skin… offers the greatest resistance…” and that the high voltage of a fence controller breaks down the skin resistance. But, Whitaker could not apply high voltage, at unlimited current, to his subjects to “break down” the skin resistance. Therefore, Whitaker’s experiments included wetting the hands and feet of his subjects with 20% sodium chloride solution.
With constant area, constant pressure, and wet hands, Whitaker found that the body resistance was independent of current, when current was in the range of 1 to 15 milliamperes.
Whitaker’s test set-up was comprised of a 12-volt dc source (dry cells), a potentiometer, a voltmeter, and an ammeter. The hand electrodes were No. 10 AWG wires. The foot electrode was a 14-inch square copper plate. The potentiometer was adjusted for 5 milliamperes for adults and 1 milliampere for children. The voltage across the subject was measured, and the resistance calculated.
Whitaker measured 40 adults and 47 children (aged 3 to 15). He found that, for adults, “there are no trends or relationships between the body resistance of individuals and their sex, age, height, or weight.” I have provided histograms of Whitaker’s various measurements in Figures 1 and 2.
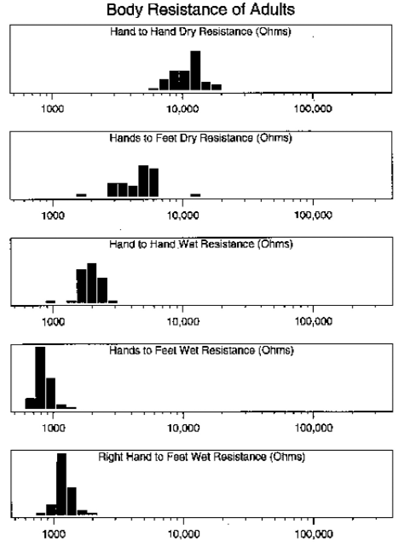
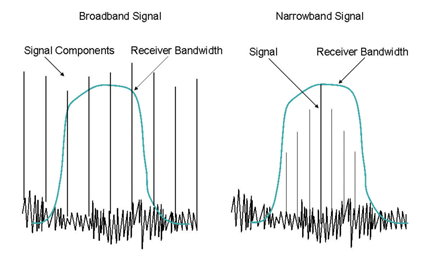
From this data, he concluded that “the lowest body resistance which might be reckoned with in connection with the electric fence application would not be less than 500 ohms.”
(In a later Bulletin of Research, this same data is used by Karl Geiges to develop the infamous leakage current meter. I will review Geiges’ work in a future issue.)
VOLTAGE
Whitaker needed to determine two factors with respect to voltage:
- If the output current is limited, does the open-circuit voltage need to be controlled?
- If the output current is not limited, what is the maximum open-circuit voltage?
Whitaker determined that the maximum safe voltage (from a voltage source where the output current is not limited) would be that voltage that did not cause bodily injury and permitted the individual to free himself from the fence.
Whitaker reports a series of tests, performed on UL staff during 1930, which, incidentally, recorded the voltage that an individual could withstand and still have voluntary control of his muscles. From this data, the minimum voltage was 20 volts rms.
Whitaker also reports of tests by International Harvester Co. where the voltage was connected to a bucket filled with water and to a hand electrode held by the subject. The subject was then asked to retrieve an object immersed in the bucket. International Harvester found that the maximum voltage for retrieving the object was from 12 to 20 volts.
Whitaker concluded that “the open-circuit voltage need not be limited provided the device incorporates inherent current-limiting features.”
However, “where no inherent current-limiting features are incorporated in the device, the maximum safe voltage… should not exceed 12. This is based upon the theory that a potential of 12 volts or less will rarely, if ever, cause a breakdown of skin resistance sufficient to permit a current flow through the body of such intensity as to cause lack of muscular control or physical injury to the person.”
FREQUENCY
Whitaker reports that “the chief difference in the physical effect of direct, as opposed to alternating current, is that the direct current does not cause contraction of the muscles to the extent associated with alternating current.”
Whitaker also notes that Kouwenhoven and d’Arsonval both found that as frequency increases, current must also increase to have the same physiological effect.
Nevertheless, Whitaker concludes that “there is no present warrant for permitting greater values of current… regardless of the frequency employed.”
CURRENT
From the same data where Whitaker determined the maximum voltage and from other data, Whitaker determined that the minimum and maximum values at which individuals retain voluntary control of muscles were about 6 milliamperes and 20 milliamperes, respectively.
Whitaker also studied the results of fibrillating current tests on dogs and sheep, as these animals’ hearts were considered to have the same response to stimulus as do humans. From the tests on sheep and because sheep have body and heart weights similar to humans, Whitaker determined that minimum fibrillating current is directly proportional to body weight and to heart weight.
Further study of sheep test data showed that fibrillation was a function of the phase of the cardiac cycle at the time the shock occurred, and a function of the duration of the shock. Whitaker found that fibrillation for a 0.1-second duration shock, required 10 times the current as for a 3-second duration shock.
Whitaker then plotted 3-second fibrillation currents for different full-grown animals as a function of body weight and heart weight. Whitaker then assumed that the minimum value of such a curve represented man. Whitaker further assumed that the minimum fibrillating current for different body and heart weights is a constant ratio, provided the shock duration is the same percentage of the heart cycle, and the shock is initiated at the same point in the heart cycle.
Using these assumptions and data, Whitaker determined that the minimum 3-second fibrillating current for 125-pounds body weight is 126 milliamperes, and for 20-pounds body weight is 31 milliamperes. (Twenty pounds is taken as the average weight of a two-year-old child.)
Using these numbers, Whitaker determined the ratio 31:126 for minimum fibrillating currents for body weights of 20 and 125 pounds.
Using this ratio, and accounting for percent of the time for a complete heartbeat, for body weight, and for heart weight, Whitaker was able to construct a “Derived Curve of Contact Time versus Minimum Fibrillating Current for a Two-Year-Old Child.” This curve approximated a rectangular hyperbola. See Whitaker’s “Graph 3” in Figure 3.
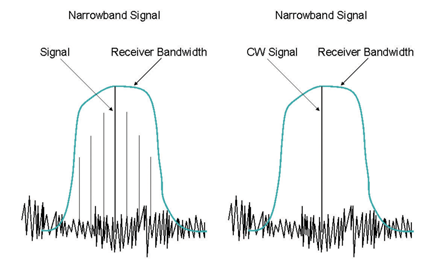
Next, Whitaker arbitrarily set the maximum current at 65 milliamperes, the maximum output at 4 milliampere-seconds, and the maximum “on” period at 0.2 seconds. This “Contact Time versus Allowable Current” curve was a factor of 6 less than the minimum fibrillating current curve. See Whitaker’s “Graph 4” in Figure 4.
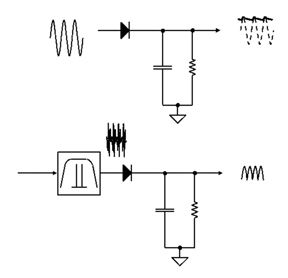
Whitaker concluded that:
- the maximum safe continuous current is 5 milliamperes, and
- the maximum duration of any current should not exceed the 4 milliampere-second curve.
OFF PERIOD
At the time of Whitaker’s research, fence controllers supplied successive shocks at about 1-second intervals. Whitaker needed to determine the minimum “off” period which would allow an individual to free himself from the fence.
UL conducted tests where a voltage was suddenly impressed on an individual, and the time to release was recorded. This test was considered an “involuntary” reaction. Whitaker noted that the time to perceive the sensation is inversely proportional to the intensity of the stimulus.
Whitaker also studied other reaction-time tests. Most of this other test data was for “voluntary” reaction to stimuli such as touch, visual, or auditory. Whitaker also noted that muscular contraction associated with dc tended to throw the victim from the conductor, while the muscular contraction associated with ac tended to be impossible to let go.
Therefore, Whitaker concluded that the “off” period for ac controllers should be 0.90 second, and for dc controllers should be 0.75 second.
FRIGHT
Whitaker also investigated whether the fright generated by inadvertent contact with a “safe” fence might adversely affect the heart or trigger fibrillation. The medical authorities he consulted were unable to predict such an event. One authority even went so far as to say that such a weak shock was not capable of causing either fright or surprise.
I suggest there are some lessons to be learned from Whitaker’s work. First, Whitaker focused on the various injuries caused by electric shock rather than compliance with standards. Of course, there were no standards at that time. Today, when we analyze a new safety situation, we seem to do so with reference to a standard rather than to the injury.
Second, Whitaker made lots of measurements, but only used the minimum, worst-case values found. This sort of pessimism is truly necessary in the field of safety. I think too often we tend to use probability and normal distributions rather than worst-case values.
Third, Whitaker makes lots of assumptions and arbitrary decisions, especially regarding animals representing humans. I suggest that we need to keep in mind that the values presented by Whitaker are not precise. Many other of the values we use in the field of safety are likewise imprecise, but we treat them as if they were precise.
Finally, I find that we don’t do such research anymore. A colleague, J. F. Kalbach, coined a term, BOGSAT, meaning “Bunch Of Guys Sitting Around Talking” to describe how a particular curve was once developed. There was no engineering or physical basis for the curve. Purely arbitrary. I would suggest that our safety standards contain too many requirements from the BOGSAT process.
ACKNOWLEDGMENTS
Jim Pierce, ETL Testing Laboratories, dropped a copy of this UL Bulletin on my desk, asking if I had read it. I had seen and read the Bulletin many years ago, so the copy just sat on my desk for many months. Eventually, I picked it up and started reading. I was impressed with the work, and thought I would review it for you.
I also want to acknowledge Henry Jones, a product safety consultant, for his comments on electric fences. Thanks, too, to Tim Kramer of Hewlett-Packard Company for preparing the histograms of body resistance.
Richard Nute is a product safety consultant engaged in safety design, safety manufacturing, safety certification, safety standards, and forensic investigations.
Photo by Tomás Fano