Challenges and Best Practices for Cell-Level Testing
The lithium-ion cell is the heart of the modern-day electric vehicle. Proper cell selection, supported by analysis and testing, can make or break a vehicle’s performance in the market. Consumer concerns of range anxiety, performance in extreme climates, and long-term reliability directly translate into brand image and warranty costs for producers.
This article will review some of the challenges and best practices associated with cell-level testing. Although the focus is on electric vehicle applications, the material presented shares applicability with other market segments in electric mobility as well as energy storage applications, both residential and grid-level.
The Cell-to-Vehicle Hierarchy
When considering an electric vehicle as a system, there is a natural hierarchy to the key components that enable the vehicle’s electrification. In simple terms, it all begins with the cells. Cells, in turn, are integrated into modules. Modules are combined to produce a battery pack. Finally, the battery pack is merged with other key components of the drive train comprising the motive aspects of the vehicle.
Although this sounds simple in concept, the reality is that any modern electric vehicle is a highly complex device fabricated from thousands of parts that is expected to perform safely in a multitude of stressful environments over the course of a relatively long lifetime and with minimal maintenance and repair. To achieve such lofty goals, a rigorous process of designing and testing the vehicle and its electrification subsystem must be faithfully followed. Not doing so risks the loss of life, property damage, and corporate liability, including financial losses and negative impacts on a company’s brand.
Note the criticality that cell testing plays in the hierarchy shown in Figure 1. The output data provides actionable information on upstream system performance. Individual cell storage and cycling aging behavior will define the corresponding module life performance, affecting the projected vehicle performance. For example, an early cell performance issue caught in the concept validation (CV) phase can drive changes in the pack, the battery management system (BMS), or even vehicle performance or warranty targets. This build-test-optimize flow is a cycle that works in both directions, from component to higher-level systems, but also “top-down,” from vehicle level down to the cell.
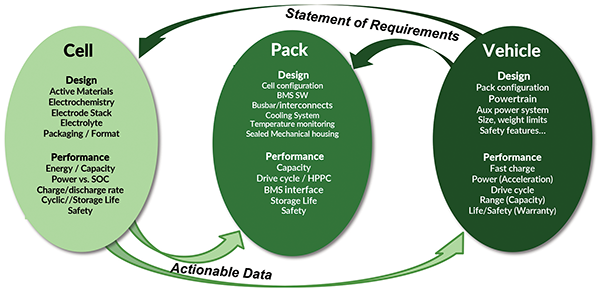
Testing and evaluation specific to the design and use of the cells can be evaluated and fed back into a design and validation loop. Think about this process as a way to complete multiple iterations early in the program to identify potential problems and validate proposed solutions for that design and future designs. This may seem complicated, but there is a structure that EV program teams use to carefully manage this maturation process.
Structure of the EV Program Development
The validation testing of a vehicle system follows a sequence of development maturity phases known as advanced product quality planning or APQP for short. APQP is a well-established methodology followed by the automotive industry (and many others) for developing a new product intended for high-volume manufacturing and under strict quality levels, schedules, and cost targets.
Figure 2 outlines the phase gate maturity levels managed throughout a program’s lifecycle. Note the high degree of variability in test validation plan durations. Beginning with concept validation (CV or A-sample) and running through process validation (PV) and the full production part approval process (PPAP), the validation duration may exceed three years. The full scope of an EV program requires an immense commitment of resources, time, and budget to successfully complete such testing.
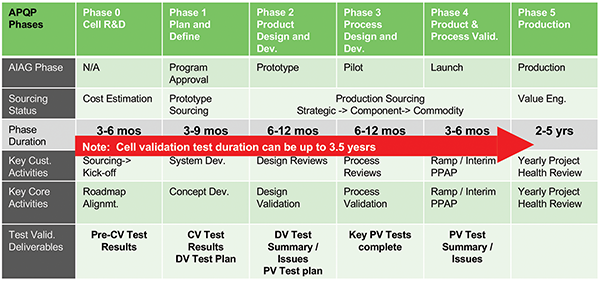
Typically, the structure of cell-level validation testing falls into seven general categories of testing:
- Beginning of Life inspection (BoL)—Basic mechanical measurements, cell OCV, 1-kHz ACR, and thickness or volume
- Characterization group—OCV, rate mapping/HPPC, DCIR.
- Environmental durability—Customer-specific corrosion, glycol immersion, or other customer-defined requirements.
- Calendar life—Storage at multiple temperatures and multiple states of charge (SoC).
- Cycle life—Various cycling at specified C-rates, depth of discharge limits (DoD), and temperatures. May include specific drive cycle profiles.
- Abuse group—Overcharge, hot box, short circuit, drop, crush, nail penetration, GBT-31485.
- Compliance—Regulatory testing per UN38.3.
Each of these testing categories is executed during an EV program’s respective phase gates. This work then proceeds in a stair-step progression from the early phases (CV) to the later phases (DV and PV). Figure 3 conveys this sequencing. Note again that the genericized timeline covers nearly 3.5 years from the early CV phase through to cell start of production (SoP).
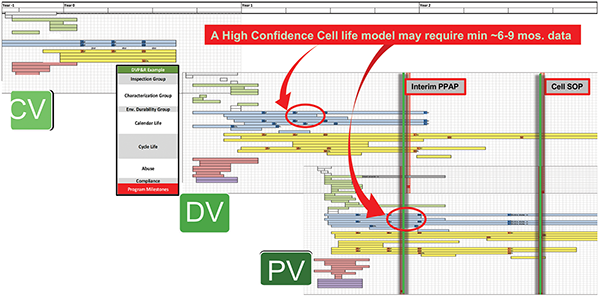
When we think about the actionable data that feeds into the system-level design, one of the most important activities is to gather enough cell data to input into a life model. A general guideline to establish a reasonable confidence life model for storage and cycling is to have 6-9 months of testing data in hand. Many research and development efforts are underway to employ machine learning and AI techniques to establish more refined modeling with a shorter duration of testing. However, this work still requires harvesting large volumes of meta-data that may not be readily available in some customer programs, for example, working with new chemistries or designs. Even if such data is available, there may still be important challenges with data quality. Examples include:
- Cell-to-cell consistency at low pre-production volumes
- Small sample sizes (cell availability may be very limited in R&D phases)
- The quality of the testing execution itself
It is this last point regarding test quality that especially drives consideration in the use of a third-party test lab. While in-house testing holds many clear benefits (control of schedules and priorities, known equipment/personnel, and in-depth knowledge of product under test), an independent lab affords improved impartiality of results, additional test capacity, independent accreditations, and enhanced credibility with unbiased external testing reports.
Approaches for Data Management
Keeping in mind the framework of the APQP process and the complexities of executing and compiling all those categories of data in Figure 3, a key best practice is to adopt a robust information management process. For EV cell testing, many multichannel test cyclers are connected to networked servers for data aggregation. The cyclers and channels are further configured with an array of chambers, incubators, and temperature-controlled rooms to maintain temperature stability during testing. Finally, a variety of other stand-alone equipment is used for periodic state-of-health (SoH) and reference performance tests (RPTs). With such large amounts of disparate data, proper data management strategy and execution are critical.
So how is this accomplished? All validation testing must have solid traceability for each individual cell serial number throughout all steps in test progression, from incoming inspection through long-term cycling or calendar aging. We have found that, where possible, the use of the complementary capabilities of an internal battery test database (BTD) and a high-resolution cloud-based battery intelligence platform (such as Voltaiq®) achieves this important goal. All cells are scanned and assigned to a single test ID code. All downstream data is linked to the test ID from that point onward. Report data can then be processed and filtered based on individual cell IDs or lots as desired. Enabling customer access to real-time data enhances communication on test status and provides a range of reporting options and advanced analytics.
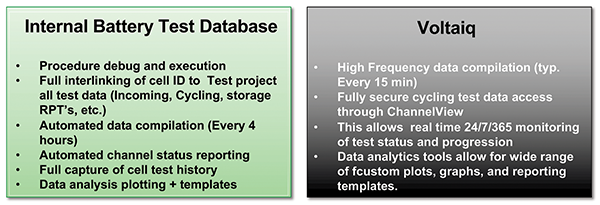
The explosion in activity in the adoption of EV technologies has led to a huge demand for third-party laboratory cell performance test capacity. Given this, planning external test needs early and carefully is important. To that end, an internal BTD provides automatic daily updates on channel availability and status (including tracking of ganged channels for higher current cycling or those blocked due to specific chamber conditions). These reported states are key inputs for providing accurate scheduling, customer guidance during the quotation, and agility during the test planning process.
Why agility? Well, in a perfect world, an overall program goal per APQP is to achieve a stable design after CV and then move on to validate through DV. But problems will occur, including unexpected process excursions, material supply variability, and unforeseen interactions in the results. Testing agents must be responsive to changes in any given test plan to meet unforeseen customer issues or requests. Using appropriately designed and implemented data management systems allows such responsiveness, permitting the quick identification of possible solutions to issues that arise during the test process.
Test Procedure Development
The cycling testers are at the center of most of the performance testing, including storage RPTs and the range of cyclic aging conditions. It is important to have staff trained and experienced with this equipment. Different models of cycler testers (Maccor, Bitrode, Chroma, Neware, etc.) have unique designs (especially system hardware capabilities) and resultant functional sensitivities. Some operational modes may be prone to causing interrupts, holds, and errors.
An example of this might be where relay switching between charge and discharge steps causes a lost current control failure, which can be mitigated by incorporating a short duration (~<1s) “phantom” 0A charge step. It is important that such sensitivities be fully understood and abated before embarking on a customer’s testing. Such knowledge comes through proper training and experience. Failure to account for such testing equipment anomalies, particularly during long-term aging studies, can have cumulative confounding effects on the overall data quality.
It is important to work with the customer at the earliest stages of the project to identify whether the customer will supply build-test procedures or need to be written by the lab based on a general outline. If they must be lab generated, planning may need to include lead time to allow for a draft-review-revise iteration before final approval. Another consideration is that some procedures are more complex and may contain advanced constructs that limit the efficacy of offline procedure reviews. Experience shows that an initial debug run with a “proxy” cell can avoid significant test problems later due to undetected programming errors.
The bottom line is that it’s best to adopt a “measure twice, cut once” philosophy. Be rigorous in procedure development, conduct de-bug testing before initiating the actual test protocol, and closely work with test equipment suppliers to identify equipment idiosyncrasies and develop appropriate mitigation strategies.
Fixturing Considerations
Whether pouch or canned prismatic, cell performance testing must have clearly defined fixture requirements. Keep in mind that a main objective for cell-level testing is to approximate application-relevant conditions. Li-ion cells will age and generate internal pressure over their lifetime. Depending on the combination of environmental and cycling parameters, this rate of swelling and gas generation will vary.
The standard practice for such testing is to use a compression fixture designed to apply controlled pressure to the cell. Typically, this assembly is composed of a 10mm thick aluminum top and bottom plate with bolts (see Figure 5). Ideally, this should be representative of the module or pack enclosure boundary conditions, including the use of identical compression pads or similar contact materials used in the pack assembly.
- Testing agents can benefit from utilizing an inventory of fixtures that cover a range of format sizes but should also have the internal capability or external vendor partnerships that support the rapid fabrication or modification of existing designs.
- Define clear fixturing requirements ASAP so that plans will factor in potential fixture lead times (i.e., target compression, cell spec sheet/size). For solid-state applications, load requirements can be much higher than standard Li-ion (75 psi vs. 8-14 psi). Confirming that the fixture assembly equipment can meet higher loading targets is important.
- Fixture assembly equipment must allow for a repeatable and reproducible load (very important as the cell may be re-fixtured multiple times as part of a test plan).
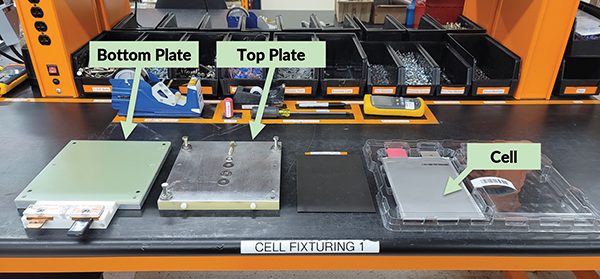
As shown in Figure 6, it is highly recommended that a calibrated load cell and hydraulic or pneumatic piston at an appropriate rating be used. Sole reliance on bolt pattern tightening may not guarantee that intended loads are achieved. Even worse, it may induce unbalanced loading across the cell under test, resulting in potentially unattributable test variances.
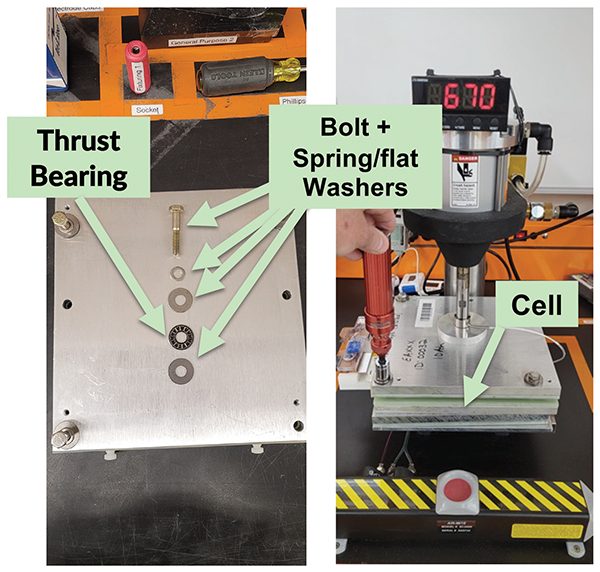
Additional points:
- Use of thrust washers allows only normal compressive force between plates while eliminating distortion effects between the interface of the bolt and plate;
- Ensure plates are clean of debris and copper tabs are not oxidized before proceeding;
- Thermocouple routing to minimize stress concentrations on cell surfaces;
- Improve redundancy and traceability by writing the request ID and cell ID on the top seal of the cell to enable easier verification that the correct cell is in the fixture;
- Check and check again to confirm that the fixture flat washers and thrust bearing are not loose after tightening.
General Lab 5-S Practices
The 5-S is a standardized approach to facility organization and cleanliness. Proper implementation leads to the smoother operation of the laboratory. This effort should be complemented with a continuous improvement versus a “one and done” mindset. Experience has shown improved safety, higher equipment availability, and lower defect and error rates can result. 5-S also creates a positive feedback loop, creating an enhanced enterprise image to customers, suppliers, employees, and management.
Figure 7 provides an overview of standardized 5-S practices. Figures 8 and 9 illustrate examples of 5-S in the general lab area and with equipment set-up practices. For example, lab benches have marked areas for needed tools and equipment, and standard environmental chamber 80-20 rack set-ups have clearly labeled cable connections, leads, and clean layouts throughout.
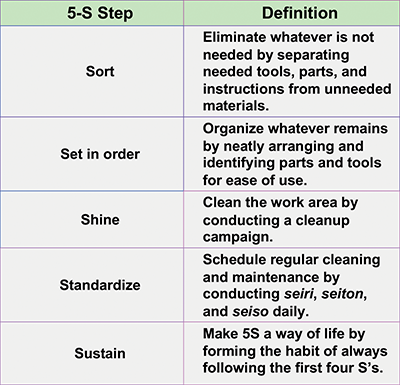
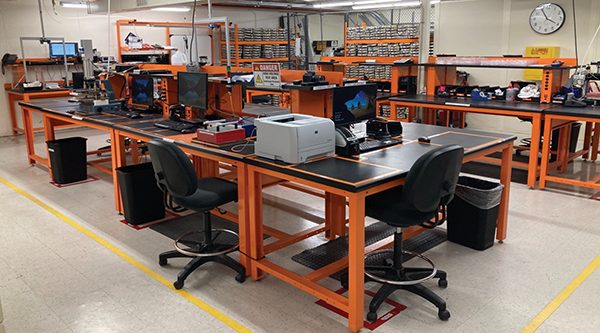
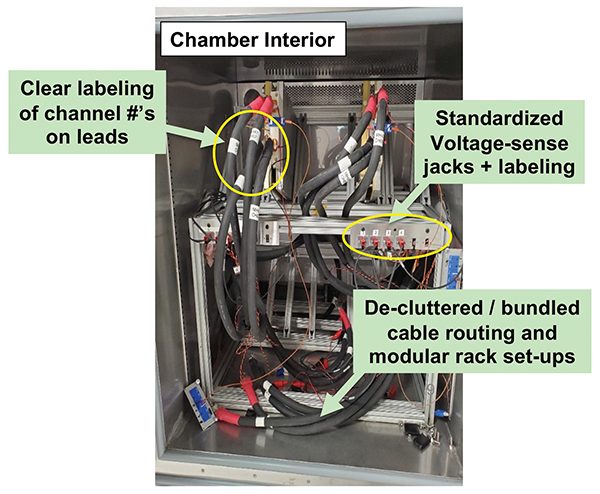
The 8-D Process and Failure Analysis
By its nature, cell validation for EVs is intended to underpin expected performance and identify failure modes. Understanding such failure modes provides more value to the product’s body of knowledge than just a series of passing tests. The 8-discipline or “8-D” process developed by Ford Motor Company provides a systematic method for analyzing failures. By carefully analyzing the failure modes, more can be learned about the intrinsic design margin and what the root cause may be. Then, appropriate mitigation steps can be taken with the cell design or with modification of certain operating conditions to reduce or even eliminate the chance of recurrence.
Several analysis tools can be used as a part of the 8-D process. The Ishikawa or fishbone diagram is one of the most commonly used structures to help organize data to arrive at a root cause (see Figure 10). Each of the main legs of the diagram documents the hierarchy of failures related to a certain category within the cell development space. Topline categories include personnel, measurement, procedure, environment, materials, and equipment.
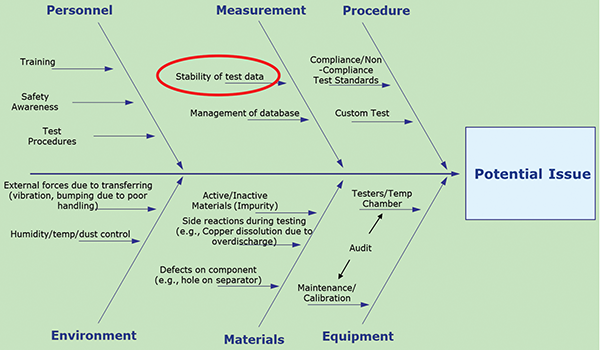
Another important part of the root-cause investigation is cell-level failure analysis. Cell teardowns are a fundamental tool that allows for gathering empirical information on the state of a cell’s packaging, internal electrode stack, and other components at critical points in the cell’s life cycle or after failures. Cell teardowns are sometimes an integrated part of a cell’s test plan, but not always.
There are generally two classes of teardowns:
- Proactive—In these cases, the customer may want to quantify warranty risks or understand and optimize cell performance; and
- Retrospective—Here, a failure has occurred, and a root cause analysis needs information on the condition of the cell and its components. Material samples may be collected for deeper forensic analysis.
UN 38.3 Considerations
Recall that the compliance testing requirements in the cell test plan must include a suite of tests for UN 38.3 transportation testing. This requirement is needed for any cells in development that fall outside of the R&D prototype classification waiver. Depending upon laboratory backlogs, this set of tests may require 1-3 months lead time to get the final certificate.
Particularly for a cell in development, suppliers should carefully review any changes to the design that could trigger the need for a UN38.3 retest. It is helpful to refer to the decision flowchart within the UN standard and the specific criteria described. It may be possible to apply a new part number with the change onto an existing transportation certificate for minor changes that do not meet the retest criteria. However, for international shipments (for example, China), customs inspection criteria can be murky. For this reason, it is critical to rigorously review the changes to clearly understand whether this constitutes a new design, thus triggering the need for retesting.
Custom Tests
Custom testing considerations can fall into a variety of validation testing types:
- Abuse—An abuse test is any test that subjects the product to non-normal stress. This can be electrical, thermal, mechanical, or chemical, to name a few. An example is custom nail penetration testing. Certain customers may specify unique penetrator details such as diameter or point dimensions, process parameters like nail speed or penetration depth, or environmental factors such as preconditioning or test temperatures. They may also seek supplemental data on response factors such as gas composition, gas volume, or pressure vs. time.
- Drive cycle—This refers to subjecting the cell to the specific charge and discharge profiles to which it will be exposed in the supported vehicular environment. Custom environmental or mechanical conditions could further supplement this. Such testing requires careful alignment of test procedures to the intended service and environmental histograms.
- Accelerated testing—Most commonly, test acceleration is achieved through elevated temperature. In the case of electrical testing, this may be further supplemented with higher charge or discharge currents. Note that many other acceleration strategies can be employed to meet a given test situation.
- Unique DOEs—Designed experiments are used to investigate design or process concerns throughout the process. Early in the design cycle, custom experimentation might include characterization tests on new cell design features. Some examples include thermal mapping vs. high current pulse discharge, Env. Soak, or Li-plating studies).
A general recommendation is to prioritize early detailed reviews between testing agents and customers on potential custom testing needs. This will help to ensure alignment regarding both capability and available capacity.
Conclusion
EV cell performance testing involves a large, multifaceted scope of planning, activities, and deliverables. A common and critical thread is rigorous and transparent communication with the customer from the earliest point in the quotation and kick-off through key milestones and final reporting. Applying lessons learned with procedure development, fixturing, failure analysis, and information management can help ensure that consistent and high-quality testing is executed. Ultimately, it is about delivering high-confidence results that can be used as actionable data to help the customer make the best decisions on a program.