Mobile phones, or “mobiles,” enjoy worldwide popularity, and one study estimates that the number of mobiles worldwide will rapidly increase to 3 billion sets.1 Analog or digital signals modulate emissions from common mobiles, their peak transmitting power can reach 2W, and their transmitting frequencies are within the ultrahigh-frequency (UHF) range: 0.3 to approximately 3 GHz.2 A hearing aid (HA) is a tiny electroacoustic device; internal wires and PCB (printed-circuit-board) traces form hidden but effective antennas, receiving the UHF signals; after demodulation, the signals may cause annoying audio-frequency (AF) noises.
To protect the hearing health of HA users, researchers in the relevant areas have been studying the effects of electromagnetic interference (EMI) on HAs. From 1997 to 2011, the International Electrotechnical Commission (IEC) created international standards specifying the electromagnetic compatibility (EMC) between HAs and mobiles; the latest version of these standards adds a specification for user compatibility and extends the high-frequency band.3 The American National Standards Institute (ANSI), from 2001 to 2011, released three versions of national standards of measurement principles and methods for EMC compatibility between mobiles and HAs. In 2010, the Hearing Loss Association of America announced the latest version of the Federal Communication Committee’s (FCC) EMC rules for compatibility between HAs and mobiles, which required mobile manufacturers to comply with these rules by a deadline.4
The book Trilogy of Magnetics describes at great length the recent development of electromagnetic (EM) technologies, including descriptions of EM theory and practice, fundamental-equivalent-circuit theory, and the properties and working areas of various EM components, and providing an overview of detailed practical examples.5 Other literature discusses the basics of EMI troubleshooting in the general electrical industries.6 Another article describes the art of optimal EMC design on multilayer PCBs and focuses on decoupling-capacitor layout. 7
However, these standard documents are comprehensive, and they target professional engineers; articles and reports on EMC-control/design techniques for HAs are rarely available. Based on fundamental radio-frequency (RF) transmitting-receiving principles and many years of practice with the EMC standards, using RF-PCB design arts, and using the new generation of EM components, this article presents the causes of EMI trouble and applicable EMC measurements and evaluation procedures and proposes several EMC-control techniques for various HA devices. Researchers and engineers in the relevant areas and hearing-care professions should benefit from reading about these topics.
Electromagnetic Interference of Mobiles to Hearing Aids
“EMI” here refers to the interference from mobiles, the unavoidable and most severe EMI in the HA environment. EM signals have a property of radiation, and the higher the frequency of a signal, the higher its radiation efficiency. A conductive wire of one-quarter wavelength can be an efficient antenna for signals of this wavelength. In the UHF range, practical impedance of an AF component can vary widely from its designed impedance; one should not ignore the roles of stray capacitance and stray inductance. For example, 0.5 cm leads of a 10-nF ceramic capacitor form 9-nH inductance.5 As a result, the electronic components have a cut-off frequency from hundreds of megahertz to a few gigahertz. For UHF signals, the coupling coefficient between wires or PCB traces is inversely proportional to their distances. Several conductive wires or PCB traces connected to AF components probably form effectively receiving antennas with a certain EMI; they even form resonance at certain frequencies, so they cause an intense output. Engineers cannot ignore these properties when analyzing the EMI of an HA.
According to W.D. Kimmel, a wire of only one-twentieth wavelength can receive EMI signals of that wavelength.6 Frequencies of the transmitting signals of common mobiles range from 800 MHz to 2 GHz, and the new generation of mobiles extends that frequency range. Roughly calculating, transmitting signals’ one-twentieth wavelengths range from 1.9 to 0.75 cm. The many PCB traces and conductive wires in HAs can reach one-twentieth wavelengths or longer. Thus, the circuits of HAs comprise many UHF antennas, which can effectively absorb nearby mobiles’ transmitting signals; the nonlinear circuits in the HAs then demodulate those signals into AF noises, sounding like chattering, quacking, or humming.
When such noises are weak, they annoy HA users; when strong, they make it difficult for HA users to understand speech. Because analog HA circuits comprise many large components and long wires, it is easy to understand why an analog HA behaves badly with EMI, even when the only interference is from a bystander’s mobile. It also is a challenge for an analog HA to suppress the EMI of the user’s own mobile. On the contrary, it is easy for a modern ITE (in-the-ear) HA to achieve EM immunity, including dealing with the interference from the user’s own mobile because the HA comprises not only small components but also short wires and traces.
Standards of HAs EMC and Compliance with EMC Evaluation
The IEC has created three standards of HA EMC: IEC 60118-13, Electroacoustics – Hearing Aids-Part 13, and electromagnetic compatibility. These standards specify configuration of test systems, measurement methods, and specifications of HA EMC. In 2011, the upgraded IEC EMC standard assigned three frequency bands: LB (low band), HB (high band), and HBE (high-band extension), and it upward-adjusted LB field strength.3 Depending on the distance between an HA and a mobile, IEC specifies two categories of the EMC: bystander compatibility, which covers those instances in which a person using an HA is near a mobile user, and user compatibility, which covers those instances in which a person wearing an HA is using a mobile. Table 1 lists the strengths of the three EMC versions of IEC standards. In compliance with the IEC standards, an essential EMC specification is IRIL (input-related-interference level). You can regard the output sound pressure of an HA exposed in a specified EMI field as SPL (sound-pressure level) caused by an equivalent AF signal inputting to the HA; this AF input level is then the IRIL of this HA. The average speech conversation has a 55 to 60 dB SPL, so when IRIL is less than 55 dB SPL, the HA under test passes the EMC in compliance with IEC standards. For better sound quality in practice, HA manufacturers always select an IRIL of much less than 55 dB—for example, 45 dB—as an excellent EMC. Table 1 also shows the widely varying field strengths between the EMC categories.
IEC 60118-13 Versions | Bystander compatibility, user compatibility | ||
Microphone mode, T-coil mode, directional mode** | |||
LB V/m (0.8~0.96GHz |
HB V/m (1.4~2.0GHz) |
HBE V/m (2.0~2.48GHz) |
|
Version 1, 1997 | 3, N/A* | 2, N/A* | N/A |
Version 2, 2004 | 3, 75* | 2, 50* | N/A |
Version 3, 2011 | 3.5, 90* | 2, 50* | 1.5, 35* |
* The first specification is for bystander compatibility, the second one is for user compatibility ** Evaluation in directional mode is conducted for bystander compatibility |
Table 1: E-field strengths of HA EMC according to IEC 60118-13
Correspondingly, ANSI has created C63.19, which includes three EMC standards for mobiles and HAs. These standards comprehensively describe the principles and methods of near-field EMC testing between devices, specify configuration of the EMC-measurement system, and detail the specifications for the EMC categories. In the three versions, the EMC specifications for HAs are the same (Table 2). In the table, the M1 rating refers to situations in which the E-field is 31.6 to 56.2 V/m or when the H-field is 0.071 to 0.126 A/m; in the specified region of a dipole antenna, a nearby exposed HA meets an IRIL of less than 55 dB; adjusting EMI frequencies and rotating HA orientations provide an EMC rating of M1 for this HA in microphone mode. Similarly, adjusting the same field strengths, the EMC rating of this HA in T-Coil mode is T1. Table 2 also lists the strengths of the E- and H-fields for M2/T2, M3/T3, and M4/T4 ratings.2 C63.19 also specifies four ratings for M1 through M4 for mobile EMC and recommends a criterion of combination evaluation of an HA and a mobile. When the HA rating plus the mobile rating is 5 or greater, the EMC evaluation of the two devices will pass; the larger the ratings, the better the EMI immunity. It is difficult for both mobile and HA manufacturers to evaluate the products together; thus, the FCC specifies that M3/ T3 is the EMC criterion of excellent HAs.4
EMC Ratings |
RF field strength input to hearing aid* (Conditions: hearing aid IRIL <55 dB SPL and hearing aid gain change <6 dB) |
|
Near-field |
E-field V/m (continuous wave) |
H-field A/m (continuous wave) |
M1/T1 |
31.6~56.2 |
0.071~0.126 |
M2/T2 |
56.2~100.0 |
0.126~0.224 |
M3/T3 |
100.0~177.8 |
0.224~0.398 |
M4/T4 |
>177.8 |
>0.398 |
* When determining the largest interference field-strength, hearing aid orientation is to be rotated continuously and transmitting frequency is to be scanned continuously |
Table 2: EM field strengths of near-field EMC complying with ANSI C63.19
Near-field EMC for the ANSI and user compatibilities of IEC standards generally have the same purpose. However, IEC emphasizes the stability, repeatability, and anti-radiation security of a measurement, and ANSI emphasizes an approach to practical near-field situation; thus, ANSI specifies using a dipole antenna for the EMC-rating measurement, and IEC selects a GTEM (gigahertz-transverse-electromagnetic) cell for the user-compatibility measurement. Theoretically, a near field of an EM wave is within a region in which the distance between a test point and the antenna’s center is less than one wavelength; the distributions and phase relationship of an E-field vector and an H-field vector are more complex than that of a far-field EM wave. The GTEM cell waveguide generates far-field EM waves, simulating near field only at strength level but having good repeatability. Although both authorities have created regulations for their own methods and specifications, both recommend the measurements of the other party. ANSI gives a table G.2 of EMC ratings versus E-field strengths, whereas both standards assure an equivalent HA immunity with both measurements. 2 (Table 3).
EMC Ratings |
RF field strength of GTEM cell (Conditions: hearing aid IRIL <55 dB SPL and hearing aid gain change <6 dB) |
Near-field |
E-field V/m (continuous wave) |
M1/T1 |
14.1~25.1 |
M2/T2 |
25.1~44.7 |
M3/T3 |
44.7~79.4 |
M4/T4 |
>79.4 |
Table 3: Equivalent ratings of ANSI C63.19 when using an IEC GTEM cell
In the IEC-recommended EMC-test system, a computer acts as the test center, automatically measuring IRIL data and printing and saving transmitting frequencies and E-field strengths and all the test results.3 For measurement accuracy, the background noise of the test system must be 10 dB lower than the minimum EMI-caused HA output noise. The calibration method of the IEC or ANSI measurement is relatively complex. A calibration file used in Table 1 always contains the information: the tolerance of E-field strength in the GTEM cell, the test frequency, the frequency step, the output of RF generator, and the gain of the RF power amplifier. When one tests an HA with the IEC test system, the computer invokes an EMC-calibration file to control the radio frequencies and field strength to work in compliance with IEC 60118-13 standard.
The following equation provides a simple way to calculate the IRIL:
IRIL(f)=HA output (f, 1 kHz)-HA gain
where f is the frequency of RF signal in the GTEM cell, and 1 kHz is the modulation frequency of the RF carrier. In compliance with IEC 60118-13, the EMC measurement has the following specification:
- The LB test includes 21 frequencies and a step of 8 MHz; the HB test requires 44 frequencies and a step of 14 MHz; and the HBE test requires 26 frequencies and step 20 MHz.
- The HA is set in RTG (reference test gain), gain test is at 55 dB input and 1 kHz, and all the advanced features of the HA are set at “off.”
- Despite which type of HA is under test, no object in the GTEM cell can affect the field strength. The orientations of the HA are 0, 90, 180 and 270° along the E-field axis.
- The hooks of BTE (behind-the-ear) HAs must connect to the 2CC coupler of an AF processor for EMC test through a 50-cm-long, ID 2-mm polyvinyl chloride tube. ITE HAs require an additional rubber “bell” on a stethoscope, to connect to an end of the tube to adapt to the
HA canal. - The gain of the HA in the EM field must change by less than 6 dB.
In compliance with the IEC standards, the test must use an HA in omnidirectional, directional, or T-Coil mode. A few methods are available for testing the gain in T-Coil mode. A simple one requires averaging, in the RTG, two gains measured with 10 and 31.6 mA/m inputs at 1 kHz and using the mean as the gain of the T-coil at 55 dB input. Place an HA into the GTEM cell and test it once for every 90° rotation along E-field axis. After obtaining the largest IRIL at each orientation in a frequency band (Figures 2 through 4), find the largest IRIL of the four orientations is the IRIL of the band. After obtaining the largest IRIL of the LB, HB, and HBE, the largest IRIL represents the maximum IRIL in at least one mode. If the maximum IRIL data in all modes is less than 55 dB, the HA just passes the evaluation of IEC EMC. The test-field strengths of user compatibility are much higher than those of bystander compatibility (Table 1). Thus, if an HA passes user compatibility, it must pass bystander compatibility; otherwise, a measurement of bystander compatibility is necessary.
Taking into consideration the measurement methods in the IEC and the ANSI standards, major HA manufacturers have all established their own EMC laboratories to measure their own products. Although IEC specifies strict regulations for IRIL measurement, in practice, the manufacturers have difficulty obtaining consistent IRIL results with the standards. Delta Laboratory once cooperated with six major manufacturers to compare the tested IRIL data and calibrated each of their EMC-test systems to determine whether they complied with IEC 60118-13. 8 However, they obtained IRIL data that showed a greater-than-10-dB variance from each other. After analyzing these measurements, the Delta Lab determined that the inconsistency of their RF-transmitting-equipment outputs was causing the large variance. Currently, manufacturers place more trust in the Delta data and use it as their EMC specification. In addition, after a manufacturer achieves the IRIL measurement of an HA, following 60118-13, the manufacturer can follow Table 3 to see whether the HA has achieved an ANSI rating, for example, an M4/T4 rating when its IRIL is less than 55 dB and E-field strength is 80 V/m.
Techniques and Effectiveness of EMC Control of HAs
Reduction of Impedances of PCB Traces and Conductive Wires
Generally, a ground in circuits returns signal currents; engineers can use it as a reference ground to short out interference and noise. However, an improperly designed ground circuit becomes an RF antenna and receives interference. Improperly grounded components and overlong traces and wires are the most common causes of HA EMI troubles. When beginning PCB design, designers can easily solve such troubles and achieve twice the results with the half effort. Engineers design PCB ground traces to as wide as possible; if they have enough room, engineers can design traces as ground straps, nets, or islands (Figure 1). Many vias connect the analog grounds of each layer of PCB, and the vias also connect with the battery ground to form a stable reference ground. In other words, some regions of the ground have equal potential. The ground wires from the PCB to the external devices are as short as possible; designers can even replace a wire with two wires twisted together to ground the EMI with less impedance.
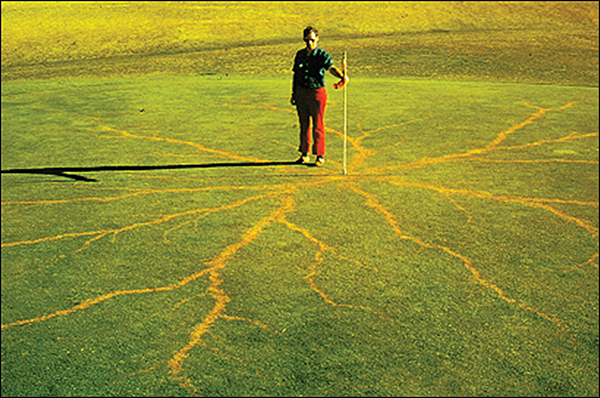
SMD (surface-mount-device) components have few stray parameters, and designers incorporate them when possible with traces as short as possible.7 HA designers always select multilayer PCBs for HAs. They arrange the ground traces, including straps, islands, and nets, on the front and rear layers and arrange the AF-signal traces on the inner layers. This approach not only shields the AF signals from the external EMI, but also reduces the ground impedances. Figure 1 shows an elaborate layout of the front and rear layers of an HA PCB. It has ground nets, islands, and straps. The inner layers have relatively more AF-signal traces. This technique adds no EMC components and is an essential technique for controlling EMC. An EMC evaluation of a BTE HA found an HB IRIL of 62.8 dB, far off the IRIL criterion. The PCB, however, had four long and thin ground traces. Modifying them into thick ground straps resulted in IRILs of 54.5 dB, an 8.3 dB improvement, enabling the BTE to pass the evaluation.
UHF Capacitors and Shielding Wires
Troubleshooting may confirm that the EMI comes from the components outside the PCB. In those cases, the above-described EMC-control technique may not apply. For example, when an RIC (receiver-in-canal) HA did not pass EMC evaluation, designers connected its separated receiver to the HA body with twin 3.5- to 5.5-cm-long wires. After measuring, we verified that the twin wires of the HA effectively received EMI in the LB. We solved the problem by connecting two UHF capacitors between the ends of the twin wires and the ground traces. The new generation of UHF capacitors comprise multilayer ceramics and SMDs and feature working frequencies as high as a few gigahertz. Such capacitors have a low Q (quality factor) but can behave with wide frequency response. The size of UHF capacitors is standardized; codes 0201 to 0603 are suitable for HAs. In this approach, we used two 100 pF capacitors with 160 kΩ impedance for an AF signal of 10 kHz and 2 Ω for a UHF signal of 0.8 GHz. Thus, the capacitors have no loss for AF signals and play a short-circuit role in UHF EMI. The grounding procedures of the capacitors should follow the previously designed technique.
Figure 2 shows the HA’s IRIL before and after the addition of capacitors. We measured this midpower RIC HA in compliance with IEC 60118-13, 2011. The figure provides the EMC evaluation of LB only because the HA had passed the EMC evaluation of HB and HBE. The horizontal solid line in the figure represents the EMC criterion. The IRIL of the HA at 0° orientation (H0) in the GTEM cell is the dashed curve; 90° orientation (H90), dotted and dashed; 180° orientation (H180), long dashed; and 270° orientation (H270), dotted and long dashed. The figure shows that, without capacitors, the maximum IRIL is 55.3 dB at 270°; with capacitors, the maximum IRIL is 45.7 dB at 0°; thus, the IRIL is 9.6 dB better.
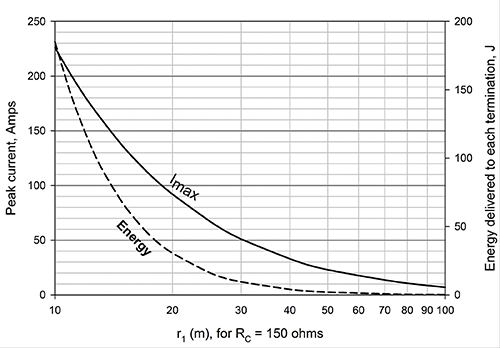
You can also effectively solve such EMI trouble with shielding wires. The two core wires carry the AF output signal; one end of the shielding skin wire connects to the housing of the receiver, and the other end connects to PCB ground. Use your judgement in selecting the trace ground of the PCB; you can try connecting the skin to a few ground spots and then determine those that behave best.
UHF Chokes
Troubleshooting confirms that the EMI comes from components outside that PCB and that UHF capacitors cannot suppress EMI from, for example, the lack of properly implemented ground traces on the PCB. In this case, designers can use UHF chokes to suppress the EMI; their working principle is to absorb the EMI and to convert it into heat energy, so there is no need to ground the EMI. The new generation of UHF chokes can work at frequencies as high as a few gigahertz, and designers can use just a few of them to meet the requirements of the PCB layout. Their cores are made of nickel-zinc ferrite, and their Q is a bit less than 3; they thus have a wide-impedance-frequency response. A UHF choke comprises two wiring coils in parallel, and it can connect into the circuit in common mode or differential mode. Such options enable the inductance to suppress the EMI, and the reversal H-fields of the currents of the two coils cancel each other, further suppressing EMI. Specifications of the chokes include inductance, DC resistance, and impedance-frequency response.
UHF chokes are a bit bigger than CHF capacitors. Designers must implement the traces for the chokes following the initially described technique. For example, consider a super-power BTE HA that did not pass the EMC evaluation. After measuring and analyzing, we concluded that the EMI came from the directional microphone’s two approximately 2-cm-long signal wires. Because the EMI currents in the two wires flowed in the same direction, using the common mode was preferable. The choke connects from the signal-wire ends to the PCB traces.
Figure 3 compares the IRIL curves of the HA in HBE with and without the choke (top and bottom, respectively). The maximum IRIL in the HA without the choke is 67.5 dB; the maximum IRIL with the choke is 51.3 dB. Thus, this approach improves the IRIL by 16.2 dB and enables the BTE HA to pass EMC evaluation.
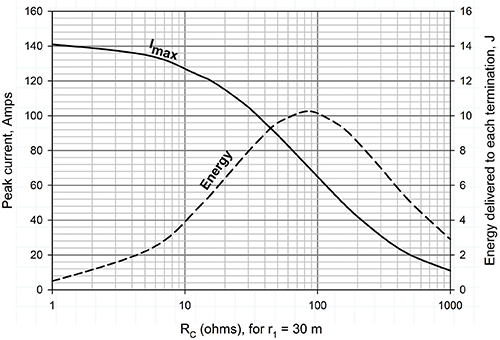
RF-Shielding Films
Troubleshooting may fail to reveal the source of EMI; alternatively, the previously described EMC techniques may provide insufficient EMI suppression. In these cases, another approach shields the entire HA circuit. For example, one could plate the HA shell’s interior surfaces with metal coating. Although this approach is effective, it can cause other troubles, such as metal corruption or the possibility that AF components could touch the coating, for example. As a result, designers have not widely adopted the plating approach. In the past few years, some EMC-component manufacturers have developed UHF-range Fermifilm shielding material, which can adhere to the interior HA shell to shield external EMI. This film exhibits good conductivity and reflection; the effectiveness of its shielding has almost no relation to the film’s thickness, which can be in angstroms. Thus, one need neither make the HA larger nor modify its mechanics. Also, you need not worry about Fermifilm’s touching the AF components or corrupting the metal. In an experiment several years ago, many Fermifilm products performed well.
Figure 4 shows EMC effectiveness of a midpower, full-digital BTE HA with a Fermifilm UIP 7-wrapped PCB unit. The top and bottom charts show the IRIL curves of the HA in the HB field without and with the film, respectively. These curves comply with IEC 60118-13 2004. The Max IRIL in the top chart is 70.8 dB; the Max IRIL in bottom chart is 38.2 dB, an improvement of 32.6 dB, enabling it to pass the EMC evaluation. The film also shielded the slots of the HA shell; otherwise, the EMC performance would have been less perfect. The open slots, depending on their size, can cause EMI to leak in. Slots with less than one-twentieth wavelength in this case can undergo leaking. A modern BTE has a few slots on top of its shell, thus limiting slot leakage. You can assemble the wires in the shell as far from the slots as possible to avoid the leakage of EMI. This technique results in excellent EMI suppression and eliminates most concerns about the EMC controls when designing PCBs. However, this approach is expensive.
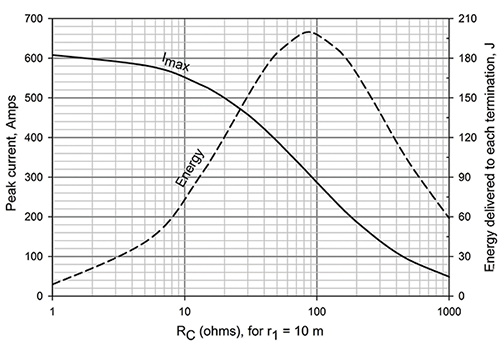
Ferrite Beads to Control EMC
The new generation of UHF ferrite beads can work at frequencies as high as a few gigahertz and behave like UHF resistors; they are tiny, flexible, and inexpensive.5 You can insert a ferrite bead into a PCB trace to intercept any EMI; the bead need not connect to ground because it converts the EMI into heat energy. When using one UHF capacitor does not sufficiently decrease EMI in a PCB to the immunity, you can add a UHF bead to work with the capacitor as an R-type filter. In an experiment, placing an R filter on an HA PCB proved more effective in suppressing EMI than using a capacitor, depending on the grounding. This approach requires slightly more PCB room, so you must finely modify the layout.
Conclusion
Today’s mobile phones cause unavoidable interference to HAs. In the mobile transmission, an HA comprises many hidden antennas of different frequencies; after demodulation, the mobile RF signal causes the HA user to hear an annoying noise. IEC and ANSI have created the standards for measurement methods and specifications for the EMC evaluation of HAs. This article discusses compliance with the standards, the EMC laboratory establishment, the operation procedures of computerized test systems, the settings of a tested HA, and the IRIL data acquisition, which are essential topics for evaluating HA EMC.
To achieve HA immunity to mobile EMI, this article also proposes several techniques of HA EMC control, including:
- Minimizing ground impedances of traces and wires: This essential technique is economical and efficient for designers starting the PCB layout of an HA.
- Using UHF capacitors and shielding wires: This technique can effectively decrease or block the EMI outside the PCB; the use of the shielding wires requires no extra room. The approach is suitable for applications in which the ground traces have very low impedance.
- Using UHF chokes: These chokes work at the frequencies as high as a few gigahertz, and they need not connect to ground traces. Exploiting the options of their common and differential modes enables further suppression of EMI. However, the approach requires a little extra PCB room.
- Implementing UHF Fermifilm. The film conducts UHF signals, isolates AF signals, adheres to the inside of an HA shell, and need not connect to ground. It has no effect on PCB layout and provides satisfactory EMC, but it is expensive.
- Applying ferrite beads: This approach is simple and flexible when solving EMI problems; it allows the implementation of individual beads or beads in combination with a capacitor; however, it requires extra room on a PCB.
This article provides some practical examples that show the effectiveness and availability of these techniques; implementing them will enable HAs
to meet the requirements of the of IEC and ANSI EMC standards.
Acknowledgements
Tables 1, 2 and 3 in this article have been adapted and reprinted with permission from IEEE, Copyright IEEE 2011.
References
- Seabury, D., and B.J. Hill, “Hearing Aid Compatibility (HAC) and Wireless Devices,” Hearing Review, Vol. 4, 2007, pp. 98-106.
- ANSI Std., American National Standard Methods of Measurement of Compatibility between Wireless Communications Devices and Hearing Aids, ANSI C63.19, May 2011.
- IEC Std., Electroacoustics – Hearing Aids-Part 13: Electromagnetic Compatibility (EMC), IEC 601118-13, June 2011.
- FCC, Consumer and Governmental Affairs Bureau, Hearing Aid Compatibility for Wireless Telephones, Hearing Loss Association of America, August 2010.
- Brander, T., A. Gerter, B. Rall, et al. Trilogy of Magnetics, Ed. 4, Wurth Elektronik, Waldenburg, Germany, 2010.
- Kimmel, W.D. and D. D. Gerke, “Basics of EMI Troubleshooting,” In Compliance, 2015, Annual Reference Guide, pp. 94-98.
- Archambeault, B., B. Zhao, K. Shringapure, et al. “Decoupling Capacitor Design on PCBs to Minimize Inductance and Maximize EMI Performance,” In Compliance, May 2015, pp. 39-42.
- Kristensen, A.G. EMC Round Robin Test – Based on IEC 60118-13, Delta Technical-Audiological Laboratory, Denmark, A900309, August 2010.
Xubao Zhang received his doctorate in electronics from Xidian University and was a postdoctoral fellow at McMaster University. He has been an EA and an EMC engineer with Unitron Canada and is the author of one book and more than 40 articles. Contact Zhang at xbzwdl@yahoo.com.