Radiated emission measurements are required worldwide as one type of EMI measurement to demonstrate product compliance with regulatory requirements. In addition, such measurements are very often performed to evaluate prototypes of products, subassemblies or design changes during the product design phase with the goal to achieve compliance before products are marketed.
In either situation a receiving antenna as a transducer of the measurement system is used to measure the electric or magnetic field strength at a certain distance from the equipment under test (EUT) and using a defined test procedure. For compliance testing such antennas must meet certain specifications as well as calibration requirements. Whereas the antenna types to be used are obvious to most EMI test laboratories the calibration requirements are known to a much lesser degree and are often felt to be difficult to determine. The situation is compounded further by the complexity of applicable antenna calibration standards and the interaction between the test laboratory and the antenna calibration laboratory.
This article identifies the key antenna specifications, reviews the significance and application of antenna factors, briefly describes the calibration requirements and defines the content of an adequate calibration request for antennas used for radiated emission measurements.
Introduction
The very basic measurement system for radiated emission measurements consists of an antenna, a cable which connects the antenna to the measuring instrument and the measuring instrument itself, as shown conceptually in Figure 1 (top view). This setup is used for measurements of the electric field strength of an EUT in the frequency range 30 MHz to 1 GHz in accordance with commercial EMI standards like ANSI C63.4-2014 [1] or EN 55011 or EN 55032.
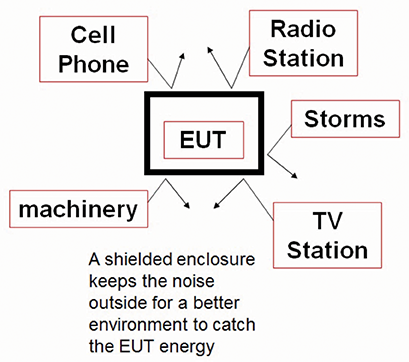
These standards also require that measurements are to be performed over a conducting ground plane. The actual test environment, which the ground plane is a part of, can be an Open Area Test Site (OATS), a weather-protected OATS or a semi-anechoic chamber. Any test environment chosen for such measurements must be validated by determining its Normalized Site Attenuation (NSA) values in the measurement axis that is used for EuT measurements. A test site yielding NSA values of less or equal to ± 4 dB (relative to the theoretical normalized site attenuation for an ideal site) in the frequency range 30 MHz to 1 GHz is deemed suitable to conduct radiated emission measurements [1,4].
The NSA determination must follow a specified procedure and setup which involves the use of two antennas. For details see standards ANSI C63.4-2014, Annex D and CISPR 16-1-4 (2010), Clause 5. Furthermore, CISPR 16-1-4 Amendment 1 (2012) Clause 5.4.4 offers a second test site validation method: The Reference Site Method (RSM). This method accounts for mutual coupling of the antennas, antenna radiation patterns and near field effects, which can have a significant influence at a 3 m test distances. Since this site validation method does not rely on the use of antenna factors to determine the test site performance the method is inherently more accurate than the NSA method.
In addition to requirements for the test environment specifications do exist for the measuring instrument which can be an EMI receiver or spectrum analyzer as well as the antennas. These specifications must be met for instruments and antennas to be used in radiated emission measurements. Requirements for measuring instruments are called out in ANSI C63.2-2009 and CISPR 16-1-1 (2015). Requirements for antennas can be found in ANSI C63.4-2014 and CISPR 16-1-4 (2010). It is to be noted that no equipment specifications do exist for cables or additional components like attenuators or preamplifiers. It is up to the laboratory to decide on their requirements.
Antenna Specifications
Several EMI standards call out antenna specifications. The ones discussed in this context are ANSI 63.4-2014 [2] (applicable to emission measurements in accordance with FCC Rules like CFR 47 Part 15.b) and the basic standard CISPR 16-1-4 [4] (which supports measurements based on CISPR product standards like EN 55011 or EN 55032). The main antenna requirements for the frequency range 30 MHz to 18 (40) GHz are summarized in Table 1.
Antenna Parameter |
ANSI C63.4 |
Comment |
|
Polarization |
Linear (4.5.4) |
Linear (4.1) |
Effectively validated through determination of cross-polarization (per CISPR 16-1-4) |
Antenna Type |
Table 1, Table 2 and Table 3 |
List of preferred antennas in Annex A |
The antenna types depends on the frequency range and the type of field to be measured |
Balance / Symmetry |
≤ 1 dB (ANSI C63.5-2006, 4.4.1) |
< 1 dB (4.5.4.2) |
Applies to symmetrical antennas (e.g., biconical and tuned dipole antennas) in vertical polarization |
Cross-Polarization |
No requirement |
> 20 dB (4.5.5) |
Applicable to log-periodic dipole array (LPDA) antennas |
Antenna Pattern |
References CISPR 16-1-4 |
Figures 18 and 10 (8.3.3.1.2.3) |
Applicable to transmit antennas used for SVSWR measurements |
Table 1: Main antenna requirements
There are additional requirements related to antennas like the 3 dB beamwidth size of horn antennas but these are related to the test distance and the size of the EUT and no specific value is called out. Some specifications for antennas used for emission measurements in the frequency range below 30 MHz do exist as well and can be found in the cited standards but are not discussed in this contribution.
Definition and Use of Antenna Factors
The purpose of radiated emission measurements is the determination of the electric or magnetic field strength arriving at the receiving antenna at a certain distance from the EUT and based on a defined measurement process. As shown in Figure 1 an antenna is connected to a measuring instrument (i.e., EMI receiver or spectrum analyzer) with a coaxial cable. The measuring instrument measures voltages frequency-selectively but does not measure field strength directly.However, these measured voltages are directly related to the field strength and hence must be converted to field strength values since the applicable limits in the standards the EUT is tested to are given in units of field strength. Common field strength units are: V/m (or dBµV/m) for electric field strength and A/m (or dBµA/m) for magnetic field strength. The relationship of the incident plane wave that illuminates the antenna (this describes the far field condition) in the direction of the antenna’s polarization ER or HR to the received voltage VR is described by the antenna factor (AF). The antenna factor is defined as follows:
for electric field strength:
If the antenna factor AF is known and the voltage VR is measured and displayed, then the measured value of the electric field strength at the antenna is:
ER [V/m] = AFelectric [1/m] * VR [V] or in logarithmic terms: ER [dBµV/m] = AFelectric [dB/m] + VR [dBµV]
The magnetic field strength can be determined in a similar way:
ER [A/m] = AFmagnetic [S/m] * VR [V] or in logarithmic terms: ER [dBµA/m] = AFelectric [dBS/m] + VR [dBµV]
It is clear from these equations that the accurate knowledge of the antenna factor AF is essential to the accurate determination of the electric or magnetic field strength.
The antenna factor includes balun losses, mismatches between the antenna elements and the balun, the the balun and the matching network as well as the electric length (also referred to as the effective height) of the antenna. Usually the insertion loss of the cable connecting the antenna to the measuring instrument is not included in the antenna factor. Therefore the cable loss Lcable (as well as the gain of a preamplifier or the attenuation of an attenuator, if used) needs to be taken into account separately when calculating the field strength. In this case the electric field strength is calculated (in logarithmic terms) as:
ER [dBµA/m] = AFelectric [dBS/m] + VR [dBµV] + Lcable [dB]
It should be noted that the antenna factor is also affected by mutual coupling to its surroundings, for example the proximity of other antennas and buildings. Furthermore, the antenna factor also depends on the angle of incidence of the field arriving at the antenna as well as the antenna impedance which is constant in free space. However, when performing emission measurements over a conducting ground plane the antenna impedance is affected by the antenna’s proximity to the ground plane as well as the orientation relative to the ground plane. The proximity and the orientation (i.e., polarization) are changed due to the required measurement process: For radiated emission measurements in accordance with ANSI C63.4, EN 55011 or 55032 for example an antenna height variation between 1 m and 4 m is to be performed for both horizontal and vertical polarization which directly influences the antenna factor.
The antenna factors used in EMI measurements to calculate the field strength are defined as free-space antenna factors which are single values at each frequency, independent of the antenna height, polarization and the distance to the EUT. The free-space antenna factor was chosen as the best compromise since the antenna factor varies quasi-periodically around the free-space antenna factor during a height scan. The effects of the antenna height, polarization and the distance to the EUT are included in the measurement uncertainty estimate for radiated emission measurements [5].
The gain of an antenna (in any given direction) is defined as the ratio of the power gain in a given direction to the power gain of a reference antenna in the same direction. It is common to use an isotropic radiator as the reference antenna in this definition. An isotropic radiator is assumed to be lossless and radiates its energy equally in all directions. This means that the gain of an isotropic radiator is G = 1 (or 0 dB). It is customary to use the unit dBi (decibels relative to an isotropic radiator) for gain with respect to an isotropic radiator. Gain expressed in dBi is computed as follows:
The numeric gain Gnumeric is the linear representation of power gain.
Sometimes, a theoretical dipole is used as the reference, so the unit dBd (decibels relative to a dipole) will be used to describe the gain with respect to a dipole. This unit tends to be used when referring to the gain of omnidirectional antennas of higher gain. In the case of these higher gain omnidirectional antennas, their gain in dBd would be an expression of their gain above 2.2 dBi. So if an antenna has a gain of 5 dBd it represents a gain of 7.2 dBi. It is to be noted that in case a single number is stated for the gain of an antenna, it is assumed that this is the maximum gain (the gain in the direction of the maximum radiation).
The antenna factor AF and the antenna gain can be related to each other using the following relationship (in linear terms):
with G being the numeric gain of the antenna with respect to an isotropic radiator and λ being the wavelength [7].
This simplified equation is only applicable if the antenna is lossless, has an impedance of 50 Ω and is used in a 50 Ω system in the far field. If any one of these assumptions does not apply then more complex equations must be used to relate the antenna factor to the gain.
Antenna gain is required for example when performing substitution measurements to determine the effective radiated power of an EUT (e.g. ANSI C63.10:2013, G.5.3).
Antenna Calibration
The accuracy of the antenna factor directly affects the measurement uncertainty of radiated emission measurements. In order to preserve accuracy and traceability of measurement results antennas are periodically calibrated. Commonly used standards for the calibration of EMI antennas are ANSI C63.5 [2], SAE ARP 958 and CISPR 16-1-6 [5]. The following discussion will focus on the requirements called out in ANSI C63.5-2006.
An EMI test laboratory must define the calibration requirements for all test equipment, including the requirements for antenna calibration. In order to request the proper calibration service the EMI test laboratory should first consider the following:
1. The EMI standards used to perform radiated emission measurements on products often call out antenna calibration requirements (e.g., ANSI C63.4:2014, 4.5.1 or EN 55025, 6.4.2.1) which are mandatory and must be complied with. If an antenna were to be used for radiated emission measurements in accordance with different standards like CFR 47 Part 15 using ANSI C63.4:2014 and EN 55025 the same antenna may have to be calibrated in two different ways which will result in different antenna factors.
ANSI C63.5:2006 includes two main methods for the calibration of antennas in the frequency range 30 MHz to 40 GHz. In principle, either of the two calibration methods can be chosen by the test laboratory. However, there are significant differences between the two methods and the antenna factors derived with these methods have a different meaning.
One method, called Reference Antenna Method (RAM), is suitable for the calibration of antennas used for radiated emission measurements in the frequency range 30 MHz to 1 GHz. This method is a substitution method that tends to yield larger uncertainties since the requirements for the antenna calibration site are less stringent and the uncertainty of the reference antenna directly impacts the uncertainty of the antenna factors of the antenna under calibration. This antenna factor is essentially a height dependent factor but due to the required calibration height (minimum 2.5 m) and required horizontal polarization the influence of the conducting ground plane is reduced. RAM requires less effort during the calibration process than the second method in ANSI C63.5:006, the Standard Site Method (SSM).
SSM does require an antenna calibration site of a defined quality which is specified in ANSI C63.5:2006. The method can be used in the frequency range 30 MHz to 40 GHz and does not rely on the availability of a suitable reference antenna. A set of three antennas is required for the basic method to determine the individual antenna factors of each of these three antennas. Since SSM involves an antenna height scan between 1m and 4 m which means the antennas are calibrated a way similar to their use during product measurements. SSM tends to yield lower antenna factor uncertainties.
In addition, per ANSI C63.5:2006 Annex G, a correction factor must be applied to antenna factors of biconical antennas that were determined using SSM. If the antenna meets certain size requirements called out in Figure G.1 of the standard and the balun of the biconical antenna is 50 Ω or 200 Ω such numerical corrections must be performed. The correction factor in Table G.1 is to be applied to obtain the free-space antenna factor for a biconical antenna in the frequency range 30 MHz to 200 MHz. This correction only applies to biconical antennas that do meet the aforementioned criteria related to size and balun impedance. For other antennas like log.-periodic antennas no correction is available in the standard and the antenna factor of SSM is to be used for product measurements.
2. If an antenna is also to be used for NSA measurements for the purpose of site validation in the frequency range 30 MHz to 1 GHz then additional requirements are to be observed. ANSI C63.5:2006 is cited in ANSI C63.4:2014 as the only permissible antenna calibration standard which in turn includes requirements for antennas used for NSA measurements.
For a pair of biconical antennas that meet the requirements discussed above correction factors are provided in Tables G.2 and G.3 of ANSI C63.5:2006 specifically for use of these antennas in NSA measurements for specific validation geometries (3m or 10 m distance, horizontal and vertical polarizations). The tables assume that the free-space antenna factor for both biconical antennas has been determined (using SSM and subsequent application of the correction factor in table G.1).
For other antenna types like log.-periodic antennas no correction for an antenna pair is available in the standard. For such antennas a measurement process is defined in Annex H to determine the Geometry-Specific Correction Factor (GSCF). The calibration laboratory will have to perform such measurements with the antenna pair that is used by the EMI test laboratory to perform NSA measurements. This means that the test laboratory must submit both antennas to the calibration laboratory since the GSCF is applicable to the antenna pair only. ANSI C63.5:2006 provides an equation how the GSCF is to be combined with the antenna factors. It is important to note that the GSCF is only applicable to NSA measurements. When one log-periodic antenna out of the pair is used for product testing, then the GSCF is not to be applied and the antenna factor for the individual antenna, derived by SSM, is to be used.
3. The number of frequencies the antenna factor is to be determined at has a direct impact on the measurement uncertainty of the emission measurement, related to the interpolation error. In general, the larger the number of frequency points the smaller the error contribution due to interpolation. Of course the actual error magnitude also depends on the antenna factor characteristics versus frequency. If the antenna factor is fairly constant or linear with frequency then fewer points are sufficient. If there are rapid changes of the antenna factor over small frequency segments then more points should be used to avoid larger interpolation errors. The number of frequency points is also affected by array size for the correction factors in the test automation software.
4. Different antenna calibration procedures yield different uncertainties of the resultant antenna factors. The consideration of the antenna factor uncertainty is important when a test laboratory is required to calculate and report (and sometimes even apply) the uncertainty of radiated emission measurements. The uncertainty of the antenna factor is a key contributor to the uncertainty calculation and lower antenna factor uncertainties do improve the overall uncertainty of the emission measurement. Antenna factor uncertainty is also significant when validating radiated emission test sites using NSA measurements. The test site must meet an acceptance criterion (calculated NSA ≤ ± 4dB). The calculation of the NSA values requires the use of the antenna factors of both the transmit and receiving antenna. Larger uncertainty values of the calibration process may lead to a test site failing the criterion – not due to site imperfections but due to uncertainties related to the antenna factors!
Preparation of an Antenna Calibration Request
As indicated in the paragraph above many different aspects have to be considered when preparing an antenna calibration request. It is to be noted that the EMI test laboratory must request the appropriate service such that the antenna can be used for the intended measurements. The calibration laboratory has no means to determine how the antenna will be used after calibration. Therefore the information in Table 2 should be included in the purchasing documentation or otherwise communicated to the calibration laboratory.
When all these elements are considered and communicated to the calibration laboratory the expected calibration service is adequately defined.
Review of Received Antenna Calibration Service
Upon return of the calibrated antenna to the EMI test laboratory the calibration service must be verified to ensure that the calibration was performed as requested. It is essential to ensure that the antenna calibration is complete and correct before the antenna is put back into service and used for product measurements. A rigorous incoming inspection will prevent nonconforming work situations which can cause additional effort in determining the impact of an incorrect calibration on measurement results. This impact can lead, under the worst circumstance, to re-test of a product and is therefore to be prevented if possible.
The items summarized in Table 2 can be used as a reference to verify that the requested calibration service was provided and is complete.
Nr. |
Information |
Comment |
1 |
Technical contact information |
If the purchasing request is not originated by a person familiar with antenna calibrations it is prudent to indicate who can be contacted by the calibration laboratory in case there are technical questions. |
2 |
Request of accredited calibration |
If an accredited calibration is requested then the calibration laboratory conforms to the traceability requirements and must include the measurement uncertainty on the certificate as well. In case no accredited calibration is requested the EMI test laboratory is responsible for establishing traceability and requesting the uncertainty of the calibration! |
3 |
Request of inclusion of accreditation body symbol |
If an accredited calibration is requested the inclusion of the accreditation body’s symbol on the certificate (e.g., A2LA or NVLAP) should be requested as well to have an obvious indication of an accredited calibration. Not all accreditation bodies require accredited calibration laboratories to place the symbol on certificates that document an accredited calibration. |
4 |
Antenna specific information |
Type, manufacturer, serial number, asset number |
5 |
Calibration standard(s) and method(s) to be used |
The actual standard, including the revision or year (e.g., ANSI C63.5:2006) as well as the calibration method (e.g., SSM). |
6 |
Frequency range of calibration |
Some antennas cover frequency ranges that are larger than the frequency range of the EUT measurement. In this case it should be clearly stated what the frequency range of calibration is. |
7 |
Number of frequency points |
As per discussion in V.3 the requested number of frequency points should be stated. |
8 |
Calibration interval |
If the test laboratory requires the documentation of the calibration interval on the calibration certificate then this is to be specifically requested. |
9 |
Special calibration requests |
If calibration parameters in addition to the antenna factor are required, like the antenna symmetry in case of biconical antennas or antenna VSWR, then these parameters are to be included in the request |
10 |
Calibration method for antenna pair |
If a pair of equal antennas is submitted for calibration for subsequent NSA measurements then the calibration method should be defined in even greater detail. It is advantageous to specify the “Equal antenna method”, as part of SSM, which allows the determination of one antenna factor for the pair, resulting in a lower uncertainty. |
11 |
Geometries and polarization for NSA measurements |
If an antenna pair is submitted for calibration to be used for NSA measurements then the geometries (i.e., distance and polarization) are to be submitted for the determination of the GSCF (see V.2). The calibration certificate should clearly state if the GSCF has been included in the reported antenna factor for the pair or if the test laboratory must include the GSCF. |
Table 2: Relevant information for an antenna calibration request
In addition, the following items should be verified during the incoming inspection:
Identification: The serial number, unique identification number (e.g. asset number or inventory number) and the Done date/Due date on the calibration certificate must match the information on the calibration label affixed to the antenna.
Accuracy: The antenna factors provided on the calibration certificate must be adequate for the intended use of the equipment. It is therefore prudent to verify the new antenna factors by comparing them to the previous factors (see Figure 2).
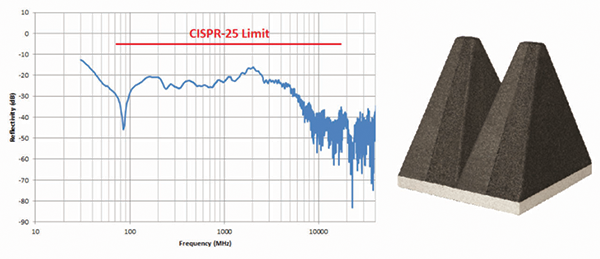
Traceability: The information which establishes traceability to national/international standards is to be verified. The presence of an accreditation symbol on the certificate, or reference to the accreditation status of the calibration laboratory can be used as evidence of traceability (through the accreditation process). Traceability is not established merely by making a statement to that effect.
Measurement uncertainty: The certificate must include an appropriate statement of measurement uncertainty, including the coverage factor.
Special instructions: If any special instructions were given to the calibration service provider for the calibration of the antenna, it must be verified that they were carried out.
Documentation of In/Out of Tolerance information: It is to be verified that information is included on the certificate indicating the condition of the antenna (e.g., “In Tolerance” or “Out of Tolerance” or similar) when received at the calibration laboratory and before shipment back to the EMI test laboratory. This will provide evidence that the antenna was
either working as intended before it was sent to the calibration laboratory or if a potential nonconforming work situation is to be considered which requires further investigation on part of the test laboratory.
Tamper-resistant seals: In case such seals are affixed to the antenna (e.g., to the body of an active monopole antenna) it is to be verified that such seals are not broken. If this is the case the calibration is deemed void.
It is good practice to evaluate new antenna factors upon receipt in the EMI test laboratory. This can be easily done using a spreadsheet, as shown in Figure 2.
In many cases the antenna factors are provided electronically, for example in a tabular format, which direct import into the test automation software without the need for manual transfer of this data.
In this case the antenna factors can also be copied into an evaluation table and be simply analyzed as shown in Table 3.
Frequency | 2009 | 2010 | 2011 | 2012 | 2013 | Max. Dev |
30 | 13.2 | 13 | 13 | 13.1 | 13 | 0.2 |
35 | 11.9 | 11.9 | 11.9 | 12.1 | 12 | 0.2 |
40 | 11.1 | 10.9 | 11 | 11 | 11 | 0.2 |
45 | 10.4 | 10.4 | 10.4 | 10.5 | 10.4 | 0.1 |
50 | 10 | 9.8 | 9.8 | 9.9 | 9.9 | 0.2 |
55 | 9.5 | 9.5 | 9.6 | 9.6 | 9.5 | 0.1 |
60 | 9.4 | 9.3 | 9.3 | 9.3 | 9.3 | 0.1 |
65 | 9.2 | 9.2 | 9.2 | 9.3 | 9.2 | 0.1 |
70 | 9.2 | 9 | 9 | 9.1 | 9 | 0.2 |
75 | 9 | 8.9 | 9 | 9 | 8.9 | 0.1 |
80 | 8.9 | 8.8 | 8.8 | 8.8 | 8.7 | 0.2 |
85 | 8.8 | 8.7 | 8.7 | 8.8 | 8.7 | 0.1 |
90 | 8.9 | 8.8 | 8.8 | 8.9 | 8.9 | 0.1 |
95 | 9 | 8.9 | 9 | 9 | 8.9 | 0.1 |
100 | 9.2 | 9.1 | 9.1 | 9.2 | 9.2 | 0.1 |
105 | 9.5 | 9.4 | 9.5 | 9.6 | 9.5 | 0.2 |
110 | 9.8 | 9.7 | 9.8 | 9.8 | 9.8 | 0.1 |
115 | 10.2 | 10.1 | 10.1 | 10.2 | 10.2 | 0.1 |
120 | 10.5 | 10.4 | 10.5 | 10.5 | 10.5 | 0.1 |
125 | 10.9 | 10.8 | 10.8 | 10.8 | 10.8 | 0.1 |
130 | 11.1 | 11 | 11 | 11 | 11.1 | 0.1 |
135 | 11.4 | 11.3 | 11.3 | 11.3 | 11.3 | 0.1 |
140 | 11.5 | 11.5 | 11.5 | 11.5 | 11.5 | 0 |
145 | 11.7 | 11.6 | 11.6 | 11.6 | 11.7 | 0.1 |
150 | 11.9 | 11.8 | 11.8 | 11.8 | 11.8 | 0.1 |
155 | 12 | 11.9 | 11.9 | 12 | 12 | 0.1 |
160 | 12.2 | 12.1 | 12.1 | 12.1 | 12.1 | 0.1 |
165 | 12.4 | 12.3 | 12.3 | 12.3 | 12.3 | 0.1 |
170 | 12.6 | 12.5 | 12.5 | 12.6 | 12.6 | 0.1 |
175 | 12.9 | 12.8 | 12.8 | 12.9 | 12.8 | 0.1 |
180 | 13.2 | 13.1 | 13.1 | 13.2 | 13.2 | 0.1 |
185 | 13.5 | 13.4 | 13.5 | 13.6 | 13.5 | 0.2 |
190 | 13.9 | 13.7 | 13.8 | 13.8 | 13.8 | 0.2 |
195 | 14.2 | 14.1 | 14.1 | 14.2 | 14.2 | 0.1 |
200 | 14.5 | 14.3 | 14.4 | 14.4 | 14.4 | 0.2 |
205 | 14.7 | 14.6 | 14.7 | 14.6 | 14.6 | 0.1 |
210 | 14.9 | 14.8 | 14.9 | 14.8 | 14.8 | 0.1 |
215 | 15 | 15 | 15 | 15 | 14.9 | 0.1 |
220 | 15.3 | 15.2 | 15.2 | 15.2 | 15.2 | 0.1 |
225 | 15.5 | 15.4 | 15.5 | 15.4 | 15.4 | 0.1 |
230 | 15.5 | 15.4 | 15.5 | 15.4 | 15.4 | 0.1 |
235 | 15.5 | 15.5 | 15.6 | 15.6 | 15.5 | 0.1 |
240 | 15.7 | 15.6 | 15.7 | 15.7 | 15.6 | 0.1 |
245 | 15.9 | 15.8 | 15.9 | 15.9 | 15.8 | 0.1 |
250 | 16.1 | 15.9 | 16 | 16.1 | 16 | 0.2 |
255 | 16.4 | 16.3 | 16.3 | 16.4 | 16.3 | 0.1 |
260 | 16.7 | 16.6 | 16.6 | 16.6 | 16.6 | 0.1 |
265 | 17 | 16.9 | 16.9 | 16.9 | 16.9 | 0.1 |
270 | 17.4 | 17.3 | 17.3 | 17.2 | 17.3 | 0.2 |
275 | 17.7 | 17.6 | 17.6 | 17.5 | 17.6 | 0.2 |
280 | 18 | 17.9 | 17.8 | 17.8 | 17.9 | 0.2 |
285 | 18.2 | 18.1 | 18.1 | 18.1 | 18.2 | 0.1 |
290 | 18.6 | 18.4 | 18.4 | 18.4 | 18.5 | 0.2 |
295 | 18.7 | 18.6 | 18.5 | 18.6 | 18.8 | 0.3 |
300 | 19.1 | 18.9 | 18.8 | 19 | 19.1 | 0.3 |
Table 3: Comparison of antenna factors for a biconical antenna
This simple approach will reveal any significant deviations of antenna factors over time which can be investigated further before the antenna is put back into service.
A sample calibration certificate for a pair of biconical antennas is shown in Figures 3 – 6.
The cover sheet (Figure 3) of the certificate includes information about the calibrated antennas (model numbers, serial numbers, assert numbers) as well as the calibration cycle (per request from the customer) and information about the status of the antenna upon receipt and before shipment back test the test house. In addition, the calibration standard (ANSI C63.5:2006) and the calibration method (identical antenna method as part of the standard site method) is clearly stated. In the note section it is stated that the corrections factors for biconical antennas have been applied as well as the correction factor for the antenna pair for NSA measurements, as required by ANSI C63.5:2006.
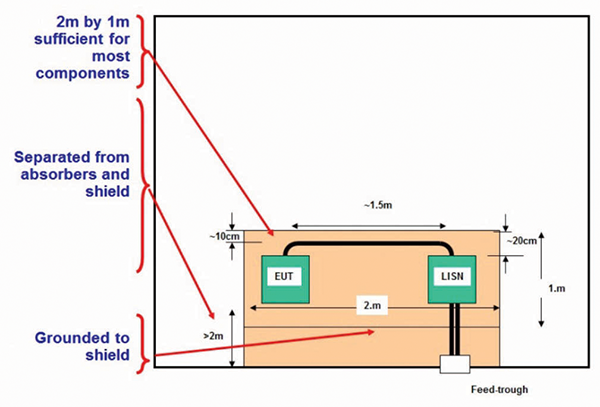
This page (Figure 4) provides information about the test equipment used to perform the calibration and references the data files that contain the actual antenna factors.
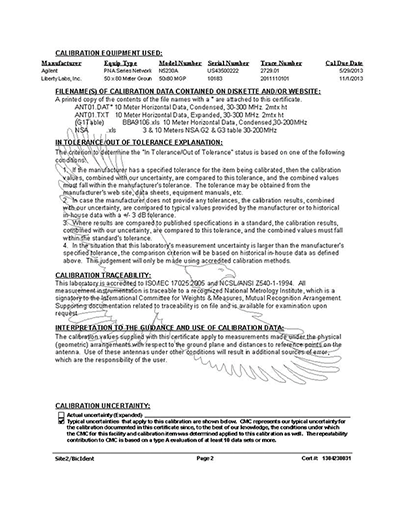
This page (Figure 5) states the uncertainty of the antenna factor based on the method used for different frequency ranges. These uncertainty values represent the expanded uncertainty based on a coverage factor of k=2 which yields confidence interval of about 95%. Therefore, a test laboratory will have to divide the reported uncertainty values by 2 in order to use these values in the uncertainty estimate for the radiated emission measurements.
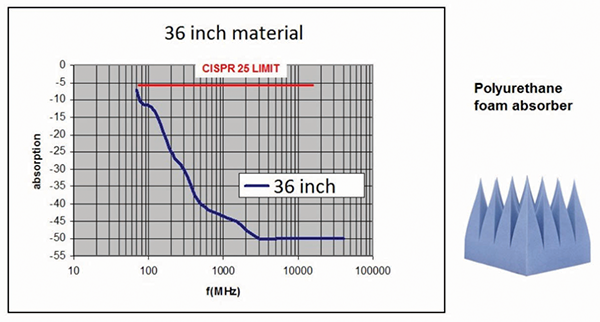
This first page of the data annex of the calibration certificate (Figure 6) includes the actual antenna factors for the calibrated pair of biconical antennas.
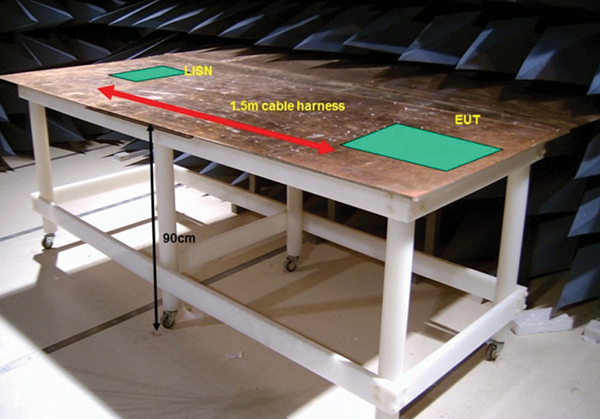
Upon completion of the calibration service review the test laboratory must update the equipment record of the antenna (e.g., change of calibration due date, inclusion of the calibration certificate) and the antenna factors themselves are to be updated in the test automation software using an established process. The correct update of antenna factors is essential – in case of errors or omission of an update none to the subsequent radiated emission measurement results will be correct!
Summary
Antennas are an essential component of radiated emission test systems. They must be carefully selected such that they meet certain specifications called out in applicable standards. Much attention is to be paid to the communication of calibration requirements for antennas. The proper definition of such requirements is the responsibility of the EMI test laboratory, not the responsibility of the calibration laboratory. Upon receipt of the antenna back from the calibration laboratory an incoming inspection is to be performed to ensure that the calibration received is complete, the antenna factors (or other calibration parameters like gain) are within the expected range and the calibration certificate properly documents the results. Such an inspection can be performed efficiently in a standardized way which will prevent the use of antennas with questionable calibration results and thus help avoid possible erroneous results of radiated emission measurements.
The author would like to thank Mr. Michael Howard of Liberty Labs, Inc. for the permission to use the calibration certificate and antenna factor data in this article and Mr. Nate Potts of Liberty Labs, Inc. for the preparation of the data.
References
- ANSI C63.4, American National Standard for Methods of Measurement of Radio- Noise Emissions from Low-Voltage Electrical and Electronic Equipment in the Range of 9 kHz to 40 GHz
- ANSI C63.5, American National Standard Electromagnetic Compatibility–Radiated Emission Measurements in Electromagnetic Interference (EMI) Control–Calibration of Antennas (9 kHz to 40 GHz)
- ANSI C63.10, American National Standard for Testing Unlicensed Wireless Devices
- CISPR 16-1-4, Specification for radio disturbance and immunity measuring apparatus and methods – Part 1-4: Radio disturbance and immunity measuring apparatus – Antennas and test sites for radiated disturbance measurements
- CISPR 16-1-6, Specification for radio disturbance and immunity measuring apparatus and methods – Part 1-6: Radio disturbance and immunity measuring apparatus – EMC antenna calibration
- Bennett, S.W., “Control and Measurement of Unintentional Electromagnetic Radiation”, John Wiley & Sons, pp. 201 – 213, 1997
- Smith, A.A., “Radio Frequency Principles and Applications”, IEEE Press, pp. 69 – 82, 1996
- Macnamara, T, “Handbook of Antennas for EMC”, Artech House Publishers, pp. 20 – 22, 1995
Werner Schaefer is a Senior Contributor to In Compliance Magazine, and the owner and Principal Engineer of Schaefer Associates. He has 31 years of EMC experience, including EMI test system and software design, EMI test method development and EMI standards development. He can be reached at wernerschaefer@comcast.net.