The field of electromagnetic interference (EMI) initially gained formal recognition quietly in 1933 under a sub-committee of the International Electrotechnical Commission (IEC) in Paris. Under the name of CISPR (International Special Committee on Radio Interference), the sub-committee was created to better understand the long-term complications that could arise from radio frequency technology. From its founding in 1820 by Morse, Henry, and Vail, radio’s popularity had exploded, becoming the must-have household appliance during the Depression. It was soon determined that intentional and unintentional RF transmissions began affecting other electrical systems, leading to a growing awareness of EMI within the electronics community. In 1934, CISPR began creating and distributing requirements regarding the recommended allowable emissions and immunity limits for electronic devices, which evolved into much of the world’s current EMC regulations.
Throughout the 1960s, 1970s and 1980s, researchers became increasingly alarmed about increased interference from electromagnetic emissions. In 1967, the U.S. military issued Mil Standard 461A, which established testing and verification requirements for electronic devices already in use in military applications, as well as emissions and susceptibility limits for new military electronic equipment.
In 1979, the U.S. Federal Communications Commission (FCC) imposed legal limits on electromagnetic emissions for all digital equipment in the U.S. As systems have become faster, smaller, and more powerful, these regulations have continued to evolve by necessity, as emerging technologies display a greater propensity to interfere with the operations of other electrical systems.
To gain a better understanding of how noise is created, avionics and aerospace engineers have investigated EMI-related issues and identified ways that new systems can be designed to minimize transmitting noise, while also being able to withstand certain amounts of noise from external sources.
Initially, most companies opted for quick, unwieldy shielded enclosure designs which were little more than minimally effective Faraday cages. More savvy researchers, from companies seeking better long-term solutions to eliminate susceptibility to EMI in their sensitive electronic devices, gravitated to a more specialized, focused approach, combining better electronic designs and layouts, while incorporating additional shielding and filtering elements when required.
The creation of multiple certification levels helped to ensure electrical systems compatibility with regard to radiated and conducted emissions and susceptibility. The introduction of these standards allowed professionals to easily identify electrical systems which could be integrated into their own assemblies without fear of EMI issues. Today, due to these stricter regulations being continuously integrated into the ever-expanding field of electronics, all types of equipment, especially highly sensitive reconnaissance, medical, and avionics equipment, are much safer from the risk of catastrophic failure due to “noisy” EMI.
EMC regulations, while extremely helpful in streamlining the creative process and assuring general design safety, do not automatically fix systems created without the forethought of extraneous radio frequency fluctuations. Many complex systems invented today are still at the mercy of EMI, due to either creating or receiving interference that can cause failure. These problems are normally solved through a combination of shielding the system from electromagnetic fluctuations, and filtering to reduce unwanted and potentially harmful energy.
EMI Shielding and Filtering
Shielding is a technique used to control EMI by blocking the transmission of radio frequency (RF) noise from a source, comparable to how lead and concrete are used as a shield from nuclear radiation. Shields can be deployed within the circuit at the RF source, the receiver or anywhere in between. In the case of electric fields where shielding is successfully implemented, the effectivity is a function of the shield material’s thickness, conductivity and continuity. Adding shielding to a cable protects the inner conductor(s) from impinging electric fields, lessening the interactive force of the magnetic field.
It is important to remember that when an electric field interacts with a conductor, it creates a voltage which appears as electrical “noise” on circuitry. When a properly grounded shield is placed around the conductor, the electric field energy is usually drained away without affecting it, thereby reducing or negating the noise. This is the basic principle that drives the common electrical shield, known as a Faraday cage.
When an electromagnetic wave traveling through space encounters a shield, several things happen:
- Much of the energy is reflected and refracted, similar to the Doppler effect on sound waves, which are partially dissipated by anything they travel through;
- Some of the residual energy is then absorbed or shunted to ground by the shield as intended, significantly reducing the magnitude of the electromagnetic wave’s energy;
- Additionally, re-reflection of energy within an enclosure must be considered in situations where a system requires thin shielding. Re-reflection occurs at the boundary on the far side of the shield material and causes bouncing of the EM wave within the system, leading to a localized spike of unwanted EM energy.
Most high frequency shielding problems are not caused by failure of the material itself to negate the noise, but by physical gaps and openings in the shield material. While shielding is a primary option for many EMI related issues, filter options can address issues associated with penetrations through the shielded enclosure, as well as the inputs and outputs of the electrical systems, typically the most vulnerable point in a shielded system.
No different than the hull of a ship, a hole at any point in the shielding system can easily lead to catastrophic failure. As the inputs and outputs are the weakest points associated with the shielded enclosures, these are the locations where filtering is most effective, due to the inherent vulnerability.
In these locations, filtering and transient suppression at the interface of the shielded enclosure is the most efficient way to protect systems from compatibility issues. By using filters and filtered connectors at the input/output interfaces of a system, design teams can eliminate the EM and RF noise from internal or external sources right at the connector interface, shunting the unwanted energy to the grounded shielded enclosure. As a result, this is the optimum location to eliminate higher frequency noise, mitigating any EMI-related problems.
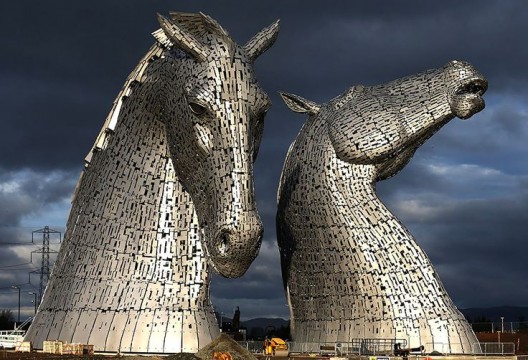
Filter Options
When considering filters and filtered connectors to help eliminate noise issues in a system, determining the best EMI solution is based on the mechanical configuration of that system. There are a multitude of filtering options, and these filters come in many different configurations, as shown in Figure 1.
Types of Low Pass Filtering Options
The filter solutions shown have multiple configurations designed to mitigate EMI problems contingent on specific system requirements. Each of these products reside within a single subset of electrical engineering, specifically, they are “low pass” filters. In other words, these filters allow the passage of lower frequency data while blocking higher frequency signals.
Low pass filters come in several implementations, as follows:
C filters are the simplest, most straight forward solution. They are built with a single decoupling capacitor from the pin or signal line to ground.
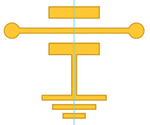
- CL/LC filters – combined inductance and capacitance filtering
CL or LC filters provide additional filtering as they are built with a single capacitor to ground and are coupled with an inductor or “choke.” This enhances both the filtering effectiveness of the decoupling capacitor as well as the filtering
associated with the impedance of the inductor.
LC filter circuits are most effective when the source impedance is less than the load impedance. CL filters are best suited in circuits when the load impedance is less than the source impedance.

- Pi filters – Capacitive and inductive filtering, (named due to the shape resembling the pi symbol, π)
Pi filters, built with two decoupling capacitors separated by an inductor, effectively trap the target noise and provide superior higher frequency
filtering performance.

It should be noted that grounding is the most important aspect of any good electrical system, and excellent ground conductivity is required for filters and filtered connectors to work properly. These designs assume that the enclosure/bulkhead to which they are mounted is a “good ground” that provides a low impedance connection path to the system ground.1
Filters must be selected based on the frequency of the noise in a system in direct correlation to the target frequency of the data signals being transmitted through the interface. The capacitance of the filter must be selected so that it does not interfere with or “clip” the edges of the data signals. Additionally, the type of filter selected must meet the frequency spectrum of the noise being addressed. In other words, does the filter only need to eliminate a narrow range in the frequency band where the noise exists, or does it need to operate more like a broadband filter, blocking a wide frequency (example: GHz) range?
Selecting the capacitance for the filter depends on calculating the -3dB cutoff frequency of the filter, which indicates the frequency where the response of the filter is 3dB down in amplitude from the level of the passband.
Below is the formula used for finding the cutoff frequency of a system:
In this formula, fc is the cutoff frequency of the filter as shown in the performance versus frequency plot in Figure 2 and R (resistance) and C (capacitance) of the equivalent circuit, as seen in Figure 3.


This information can be difficult to determine as systems continue to compound and grow for electrical engineers, let alone other specialists working in associated fields. Fortunately, most major filter manufacturers provide insertion-loss plots which clearly show the -3dB cutoff frequency associated with the various filters they offer. This data is typically presented in tabular form and is normalized for a standard 50Ohm load. Consequently, the selection of filters is made easier by reviewing the published insertion loss or filter performance data at the various frequencies for the differing capacitances.
It is important to note that chip capacitor filters behave in a less than perfect mode; they act more like a “notch” filter rather than a high pass filter due to the self-resonance of the chip capacitors. While this behavior is negligible in some applications, these notches of filtering capacity are of utmost importance, and must be understood to effectively reduce high-frequency noise in an electrical system. These chip capacitor filters have limited high frequency performance versus the predicted filter performance of an “ideal” capacitor as shown in Figure 4.
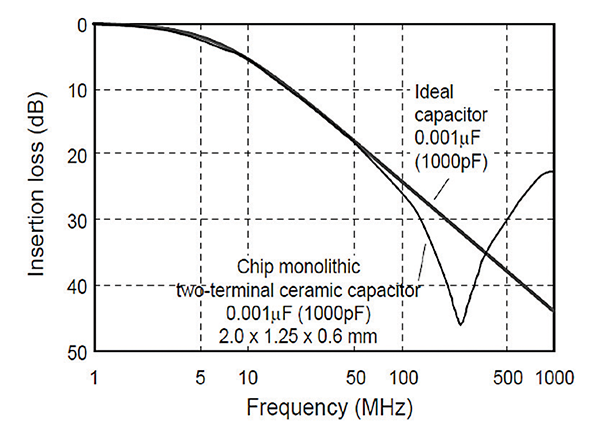
Additionally, the mechanical packaging and circuit layout of filters using chip capacitors greatly impacts the performance of the filtering. As seen in Figure 5, the equivalent series inductance directly impacts the filter performance. Design teams should take great care in the layout and conductivity of the traces used when connecting these devices to the signals and to the grounds to minimize this impact, allowing the best filtering performance achievable by the chip capacitor.
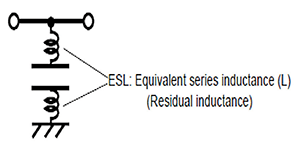
These low pass filtering devices are available in many physical forms but also come with varying performance levels. Selection of these devices is dependent upon the frequency or frequencies of the noise issues as well as the magnitude of the problem.
Figure 2 also shows the relative filter performance for the various filter types, as well as for the simple chip capacitor filters. From this data, it is evident that chip caps act like the previously mentioned notch filters, whereas C, CL, LC and Pi filters, constructed with planar arrays, discoidal capacitors or ceramic tubes, deliver better broad frequency and higher overall levels of filter performance.
Filters in Application
Armed with this basic understanding of the field and its associated components, the next step is to look at some real-time applications and analyze the filter selection process from the perspective of a specialist in EMI.
In Figure 6, notice the measured noise in a system versus the allowable limits as shown by the solid red line.
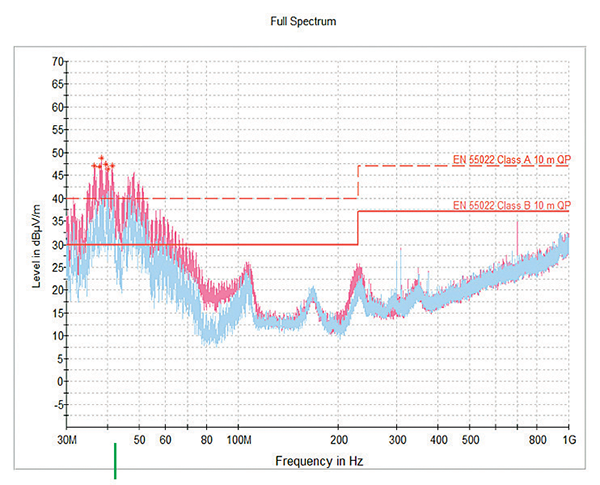
Consider the plot in Figure 7 to be the noise output of a device measured with no EMI filters, which resulted in a costly production stoppage. It is important to note that the frequencies between 30MHz and 70MHz exceed the allowable limit, meaning the design could not go to market regardless of the actual product’s success. Even though this device had a shielded enclosure, the area requires either an extremely expensive re-design or additional filtering to reduce this noise to acceptable levels.

The largest outage for this device was at 39MHz, shown in Figure 6 above with a green hash mark. Based on this observation, it is necessary to select a filtered connector that will allow the low frequency data (in this case, below 1.0MHz) to be transmitted without compromise or degradation, while filtering out the high frequency noise in the system.
Armed with this this information, and coupled with reviewing the filter manufacturer’s performance data shown in Figure 7, it can be determined that filtering in the range of 10,000pF to 30,000pF is most likely the best EMI solution for this application.
Next, it is recommended that the design team determine if a chip capacitor filter would be suitable to block the frequencies of concern, based on the low cost and high reliability of that type of filtering system. With this in mind, tests are conducted with two general system solutions, a 10,000pF capacitor filter insert and a 22,000pF capacitor filter insert.
Figure 8 shows the results of testing the 10,000pF chip cap insert. As a result, the device was found acceptable in the upper frequencies, but did not deliver sufficient filtering in the lower frequencies (between 10MHz and 60MHz) to reduce the noise below the allowable test limit.

Testing was then conducted using the 22,000pF chip cap filter insert, attempting to adjust the maximum filter performance into the lower frequency area where the noise persisted. As seen in Figure 9, performance was improved, with only small spikes at or near the noise limit in the lower frequencies. But this filter caused “ballooning” of energy into the higher frequencies around 105MHz, exceeding the allowable limit.

These test results demonstrated that generic chip capacitor filter inserts did not provide sufficient filtering. To permanently address this issue, a more specialized approach utilizing additional filtering would be required.
In order to achieve the desired results, the customer tested a specialized filter insert, designed with maximum attention to the reduction of the equivalent series inductance. This effect is achieved by mounting the chip capacitor across an isolation channel separating the pad contact area from the signal pin and the solid ground plane.
This filter insert design delivered maximum shielding effectiveness and minimized the equivalent series inductance, providing the best possible performance available from the chip capacitor while also capitalizing on the shielding already present within the system. Combined with a specialized 22,000pF filter, the filter insert achieved the results shown in Figure 10, thereby meeting EMC regulations with a cost-effective, long-term solution.
After considering the results of these tests, the device design was finalized utilizing the broadband filter (Figure 10) as there was not a lot of margin in the higher frequencies where the chip capacitors provided only minimal filtering. This specialized 22,000pF discoidal filtered connector, (a C filter built with discoidal capacitors) delivered the higher levels of filter performance as well as broader frequency performance required.

This approach improved overall EMI performance compared with other filters tested, including those of the same capacitance, by utilizing the shielding already present in the design, rather than attempting to filter the system as a “magic bullet” solution. It supplemented the parts of the system that were successful, enhancing the overall performance of the entire system, instead of treating the system as a separate entity.
Conclusion
In summary, approaching EMI noise issues is more akin to a physician approaching a patient than to most forms of remedial engineering solution testing. The initial steps involve collecting information on obvious “symptoms” of the problem. During the assessment phase, using a spectrum analyzer with a near field probe to evaluate the interior and exterior of the system can help identify the sources of noise, comparable to a doctor taking X-rays of an injury. Once a good assessment or diagnosis of the problem has been made, treatment can begin with the application of simple remedies first before evaluating more complex solutions.
The real-time example illustrated here relates back to the core theoretical issues related to grounding. The majority of all EMI problems come from improperly grounded electrical connections becoming unintended transmitters or receivers of EMI, or turning into points where EMI can leak into or out of a system. To address such problems, first attempt to resolve any grounding issues. Next, try patching the system with shielding, either on the board level or on the enclosure level to control the transmission of the noise in the system at the areas found during the sniff testing.
If issues still persist, start looking at filtering solutions, as described in this article. And don’t forget to seek the advice of a qualified EMI consultant, who can help you identify a reliable EMI filtering solution.
Endnotes
Shielding and Grounding is not covered specifically in this article. We personally recommend “While Shielding Actually Works, Filtering is Best” at www.4emi.com as an more in-depth overview.
![]() |
Robert Ydens is the Senior Founding Engineer and President of EMI Solutions, headquartered in Irvine CA. With over 35 years spent focused on electromagnetic interference technology, Ydens’ experience ranges from developing complex systems for the space shuttle program, as well as classified programs for the DOD. Since founding EMI Solutions in 1996, Ydens has grown the company into the leading WOSB in the EMI filter and filtered connector industry. He holds a BSEE degree from the University of New Mexico. |
![]() |
Bradley Ydens is an applications engineer with EMI Solutions. During his career, he has worked on a wide breadth of projects ranging from solar powered vehicles, autonomous rovers, cyber security, robotics, and EMI/RF protection. He holds a Bachelors of Science Degree in Electrical Engineering with a minor in Computer Science and Engineering from Santa Clara University. |
The authors can be reached at engineering@4EMI.com. |