Placing a target too close to an ionizer, whether AC or DC, may increase the risk of negative effects, such as localized charge buildup, electrical stress, or minor material degradation. To prevent adverse effects and potential damage, maintain an appropriate distance from the ionizer. While AC ionizers can generate higher electric fields than DC ionizers, their impact on the target must be considered in the context of the target’s impedance, size, and the ionizer’s operational frequencies. When these factors are accounted for, the potential risks posed by AC ionization to the target are not significantly greater.
Test Setup
The experimental setup consisted of two ionizers, Model 5645 MP (AC) and Model 5225 (DC) ionizing bars, suspended above a perforated table, as shown in Figure 1.
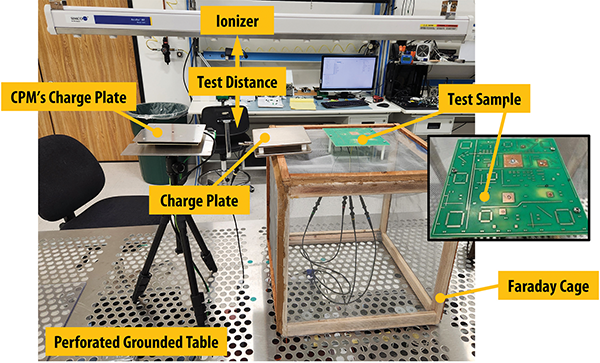
The test sample in Figure 2a was designed to simulate the exposure of targeted devices and structures of various sizes. The experiment used four pads: a 1.0‑inch square, a 0.5-inch square, a 0.25-inch square, and a 0.018 x 0.057-inch rectangle. Each pad was connected to a Lecroy WaveSurfer 64Xs oscilloscope using a 10 MW (9.5 pF) probe. A Faraday Cage was used to shield the probes from the electric field and minimize measurement contamination.
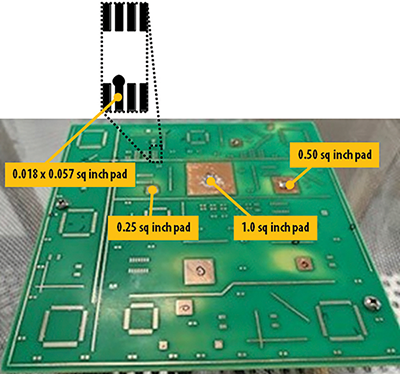
Measurements
We already understand that a DC ionizer presents minimal risk due to its nearly negligible electric field (E-Field). Therefore, our focus is on the results from AC ionization. At various distances, our test sample was exposed to two output levels from the 5645 MP bar. As expected, the electric field strength–and consequently the induced peak-to-peak voltage–decreases rapidly with increasing distance. Notably, the ionization or “pusher” frequency was not prevalent in the data. The MP (Modulated Pulse) architecture consists of a high-frequency excitation voltage (around 20 kHz) and a lower “modulation” or pusher frequency (ranging from 0.3 Hz to 60 Hz) that drives ions toward the target when purging air is not employed. Figure 3 shows the peak-to-peak voltage results for the 1-inch square test sample, with similar outcomes observed for the other sample sizes.
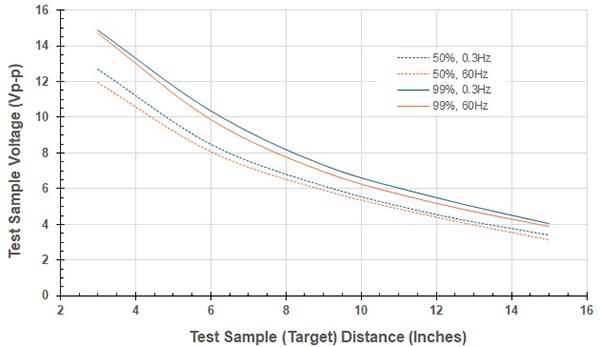
It is this excitation voltage that is responsible for inducing the voltage on our test sample, as illustrated in Figure 4. This figure shows that as the sample size decreases, the induced voltage also decreases. It is important to note that as the test sample size reduced, interference from the sampling connection increasingly affected the measurement accuracy. Consequently, obtaining a precise measurement for a 0.018 x 0.057-inch square sample became challenging.
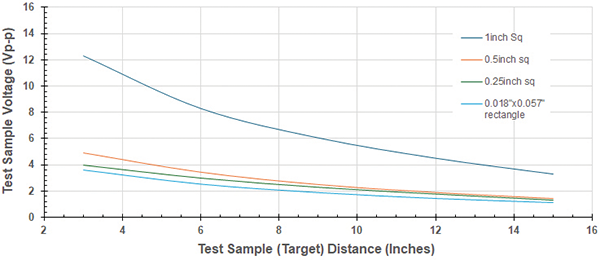
What Does All This Mean?
First, for comparison, Figure 5 illustrates the induced voltage on a standard, 20 pF 6×6-inch square, charged plate (Figure 2b). This reflects an observation when using a charged plate monitor (CPM) to evaluate ionizer performance. However, this can be misleading as typical devices would not experience such high voltage levels. Additionally, real devices have impedance, which would result in significantly lower voltage levels than those shown in Figure 4.
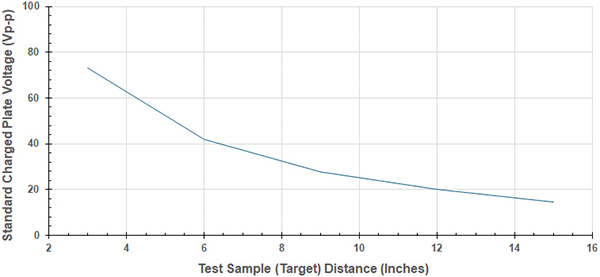
Second, all the voltages measured in this experiment are relative to a ground reference. However, in practice, a device is not damaged by Electrostatic Discharge (ESD) because of its voltage relative to ground, but rather from the interaction with nearby charged areas within the device or contact with other non-grounded objects.
It is important to note that the frequencies generated by AC ionizers are in the range of tens of Hertz, with a median wavelength of about 5,000 km. The 5645 MP also includes a 20 kHz component with a wavelength of 15 km. This means at such wavelengths all objects within the ionizer’s range are subjected to the same instantaneous voltage at the same moment. Therefore, if we compare the voltage between two objects at equal distances from the ionizer, the voltage difference (differential voltage) will be zero or near zero. Since a significant differential voltage is required to trigger an ESD event, no event can occur when the differential voltage is zero.
Consider this: Why do sparks (ESD events) occur when you place a metal object in a microwave oven? Many microwave ovens operate at a frequency of around 2.45 GHz, corresponding to a wavelength of 12.2 cm, and generate high power (about 1 kW)1. At this short wavelength, regions of high differential voltage are created. On the surface of the metal object, points only 6 cm apart experience instantaneous voltage differences, with the differential voltage corresponding to the peak-to-peak variation induced by the magnetron tube. This results in sparks jumping between those points.
In a microwave oven, any object within 6 cm experiences a significant differential voltage. In contrast, for a typical ionizer to generate a similar voltage difference, the object separation would need to be λ/2, or about 8 km (8000 meters). This is far from the case in semiconductor devices, where the separation is typically on the scale of nanometers (nm).
Third, what happens when a non-grounded object comes into contact with a semiconductor device under an operating ionizer? As the object approaches the device, it enters the same “zone of influence” created by the ionizer and experiences the same electric field as the device. This rapidly reduces the differential voltage between the object and the device to near zero, eliminating any threat to the device.
Other Concerns
During the experiment, it became clear that as the test sample was moved closer to the ionizer, additional frequencies unrelated to ionization were detected. These frequencies (characterized by long wavelengths) pose no risk but are worth noting. These “other frequencies” arise from the high voltage (HV) generation inside the ionizer, which is necessary for ion production. The HV generation (illustrated in Figure 6) typically involves either a fly-back or resonance circuit powering a step-up transformer. This creates an electric field that becomes noticeable when the device is within approximately 3 inches (or less) of the ionizer. Any ionizer, whether DC or AC, which uses high voltage generation will produce this effect.
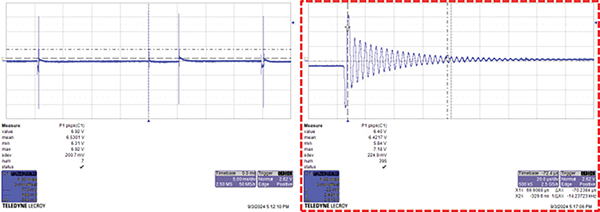
Conclusion
It appears that the frequencies generated by both DC and AC ionizers are so low (with wavelengths much greater than millimeters) and of such minimal power that they cannot produce significant differential voltages capable of causing catastrophic damage to the device. Even when other tools are introduced into the ionizer’s “zone of influence,” the resulting differential voltages remain insignificant and pose no risk of damage to the device.
Additionally, placing a target too close to an ionizer, whether AC or DC, can increase the risk of negative effects, such as localized charge buildup, electrical stress, or minor material degradation. To avoid these issues and potential damage, it’s recommended to maintain a safe distance from the ionizer.
Endnote
- “Introduction to RF Solid State Microwave Heating,” Slipstream Design.