This article describes a practical approach to the design and build of a sleeve dipole antenna mandated by a custom EMC standard. The antenna was required to operate over the frequency range of 220 MHz to 225 MHz specified in the standard. An additional requirement of having an input impedance close to 50 Ω was imposed to ensure good antenna efficiency when used with 50 Ω characteristic impedance test equipment. We begin our discussion by reviewing a symmetrically-fed half-wave dipole antenna followed by the asymmetrically driven dipole, and finally focusing on a design and build of a sleeve dipole antenna.
Symmetrically Driven Half-Wave Dipole Antenna
Symmetrically-driven half-wave dipole consists of a thin wire fed or excited at the midpoint by a voltage source. The total length of a dipole equals half-wave length. Each leg of a dipole has a length equal to the quarter of a wavelength, as shown in Figure 1 (a). Often the voltage source is connected to the antenna via transmission line, as shown in Figure 1 (b).
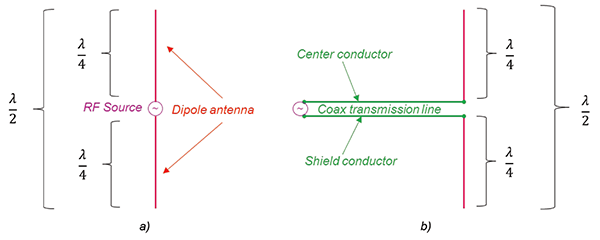
To maximize the radiated power, the antenna should be matched to the transmission line. The input impedance of the (ideal) symmetrical half-wave dipole is
(1)
The impedance of practical dipoles depends on the thickness to length ratio of its elements, with typical values of the real part in the range 60 – 70 Ω [1]. To maximize the power delivered to the antenna its length is usually shortened to make the impedance purely real. This length shortening can be performed through iterative physical length reduction and impedance measurements until the desired characteristics are obtained. This time-consuming process can be reduced through software simulations if available.
The half-wave dipole antenna is one of the simplest, yet practical antenna but its impedance is very frequency sensitive, i.e., it has a narrow bandwidth. The input impedance of the half-wave dipole can be reduced by moving the fed point off-center and the bandwidth can be increased by the addition of a sleeve, as discussed in the next section.
Asymmetrically Driven Dipole Antenna and a Sleeve Dipole
We can reduce the input impedance of a dipole by moving the fed point off-center, this is shown in Figure 2 (a). The creation of a sleeve, shown in Figure 2 (b-c) can increase the bandwidth up to more than an octave and fine-tune the input impedance [2].
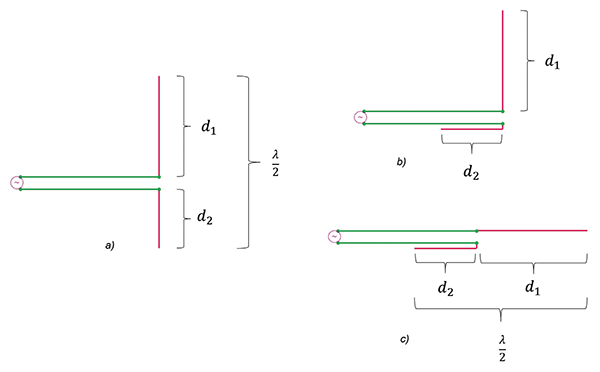
Figure 3 shows the details of the antenna connections.
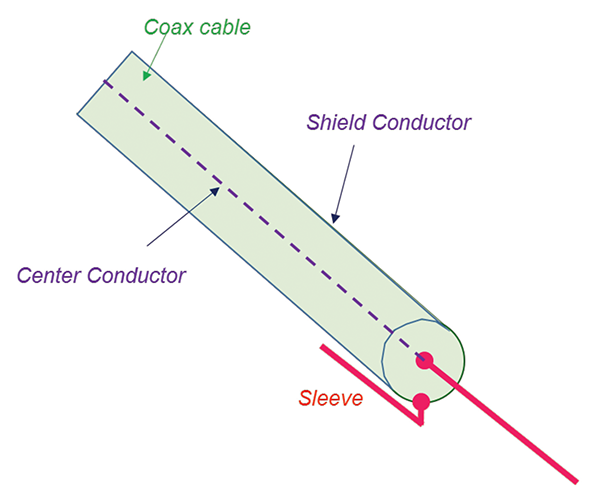
Sleeve Dipole Antenna Design
The desired operating frequency of the antenna was 220 – 225 MHz, and the desired input impedance was 50 Ω, so that it could be matched to a 50 Ohm coaxial transmission line.
A simple way to begin the design process is to start with the symmetrical sleeve antenna where both antenna elements are equal to each other, each being λ/4. This length can be determined from
(2)
where v is the velocity of signal propagation in a coax, and f0= 222.5 MHz. Subsequently one could build such an antenna and measure its response. It is safe to say that the antenna’s response would not satisfy the design requirements. The next step would be iteratively adjusting the length of the antenna elements to create an asymmetrical antenna and repeat the testing until the desired response is obtained.
Needless to say this is an ineffective and time-consuming process. With the help of a simulation software one can arrive at the proper antenna dimension in a more efficient way.
Sleeve Dipole Antenna Design through Simulation
A 3D model of a sleeve dipole was drawn in CST Microwave Studio [3]. Electrical material properties were assigned. Images of the antenna used in simulations are shown in Figures 4 and 5.
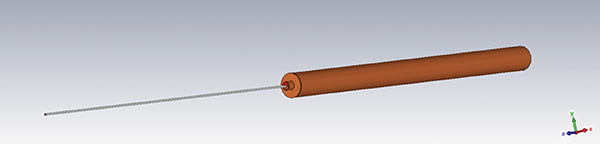
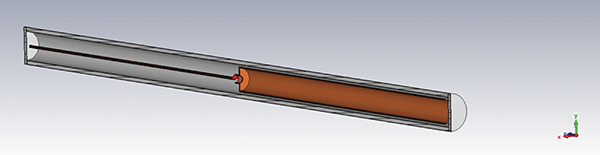
Assuming a symmetrical antenna the starting length of each arm was determined as
(3)
It is not necessary to use the exact speed of the signal propagation, as the antenna length will be determined using an optimizer program, as discussed later. The simulation was performed from DC to 300 MHz. The resulting reflection coefficient magnitude (s11 parameter magnitude) is shown in Figures 6 and 7. Figure 6 reveals that the antenna resonates at 199 MHz which is not within the antenna’s desired operating frequency of 220 MHz to 225 MHz.
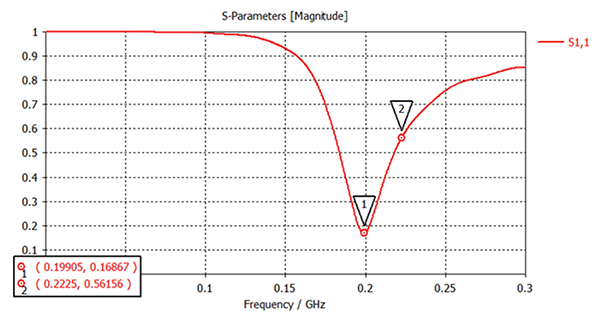
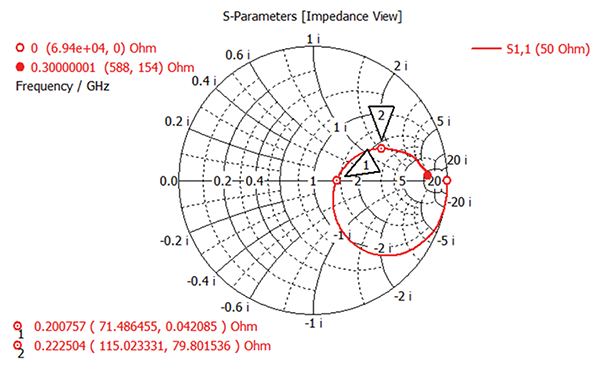
Figure 7 confirms this result by showing that impedance is real at 200.8 MHz, and equal to 71.5 Ω.
In order to determine the optimal antenna length the simulators optimizer was run with a goal of minimum S11 magnitude in the frequency range 202 – 225 MHz. The antenna element lengths were set as free parameters, not necessarily equal, in the range of 150 mm to 340 mm.
The optimizer found the optimum element lengths to be 218 mm for the tube element and 330 mm for the wire element. A plot of the magnitude of the antennas s11 is shown in Figure 8. This plot reveals the minimum magnitude at 223.5 MHz, which is in the desired range.
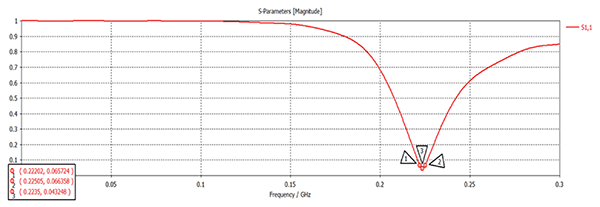
The impedance is real valued (around 54 Ω) at 223.5 MHz as shown by the Smith chart in Figure 9. It was also observed that this simulation with asymmetrical elements has a wider bandwidth than the simulation with symmetrical elements.
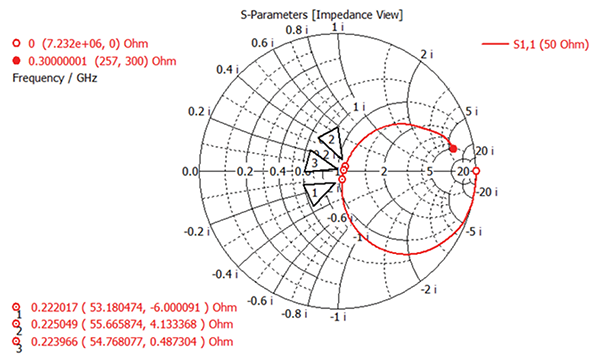
Construction and Tuning of a Sleeve Dipole
The simulation results were used to create a physical antenna shown in Figures 10 and 11. The antenna was constructed using a piece of copper pipe, copper end cap, a BNC female to solder-cup bulkhead and a length of 18 AWG solid core wire with PVC insulation. The enclosure was made from PVC pipe and end caps. The feedline is a 50 Ω coaxial cable with 50 Ω BNC male connectors. The antenna was constructed using the element lengths found by the optimizer. The wire element was 330 mm and the copper tubing element was 218 mm.

To limit the common mode current on the antenna feedline, three ferrite chokes were placed on the antennas feedline as shown in Figure 11. The sleeve dipoles reflection coefficient (S11 parameter magnitude) was measured using a vector network analyzer in the setup shown in Figure 12.
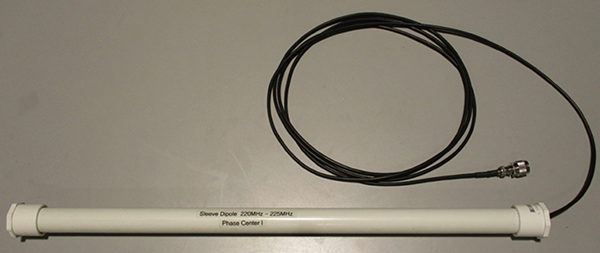
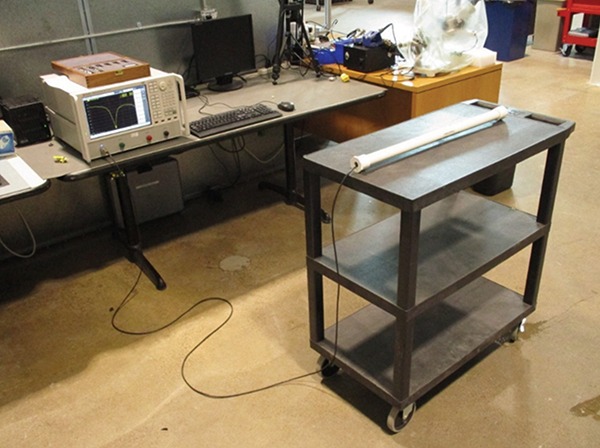
While the input impedance of the antenna requirement was satisfied (low reflection coefficient at resonance), the resonant frequency of the antenna was found to be at 210.6 MHz (instead of the target 222.5 MHz), as revealed by the plot in Figure 13.
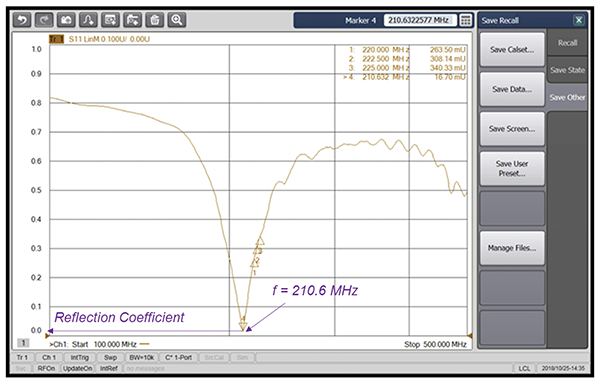
To determine the target length of the antenna elements the relationship in Eq. (4) was used:
(4)
The target lengths were determined as
(5)
(6)
Lengths of wire and copper tubing were cut from the antenna to achieve the target length. The antennas S11 parameter magnitude was measured again with the VNA, as shown in Figure 14.
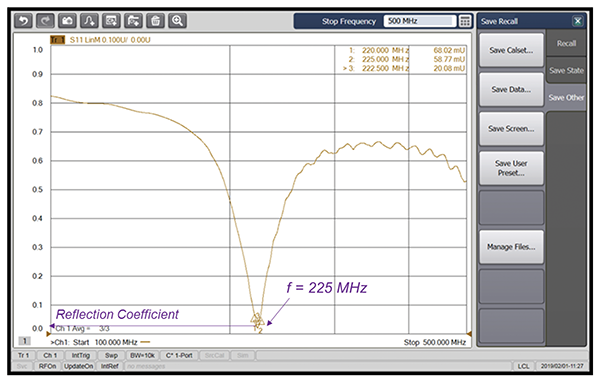
This figure reveals that both the redesigned antenna meets input impedance requirement of being close to 50 Ω and its resonant frequency is in the vicinity of 225 MHz.
References