A “battery” is the generic term for an electrochemical source of electricity, which stores energy in a chemically bound form, and which can convert this directly into electric power. A battery may be either a single cell or multiple cells connected in series/parallel configurations.
A battery cell consists of a container, electrodes (anode and cathode), separator material, electrolyte, and conductive current collectors. The container is the metal can, plastic case, or foil pouch housing of the cell. The anode (the negative electrode) is where the cell’s oxidation reaction takes place, generating electrons to the external circuit. The cathode (the positive electrode) is where the cell’s reduction reaction takes place, consuming electrons from the external circuit. The separator is a physical paper/non-woven film barrier which electrically insulates the anode from the cathode (preventing electrical internal short circuits but allowing anions/cations to freely pass. The electrolyte is the aqueous (non-aqueous for lithium batteries) medium providing the ionic conduction inside the battery. The conductive current collectors are typically the carrier metal substrates holding the anode/cathode active ingredients. For Li-Ion, these are copper foil for the anode and aluminum foil for the cathode.
Batteries are categorized as being either primary or secondary systems. Primary batteries are disposable batteries, i.e. batteries that are used once then discarded. Primary batteries are not engineered for recharging and doing so may result in an explosion. Secondary batteries, whose anode and cathode discharge reactions are reversible, are engineered so they can be safely recharged. During recharge, the “discharged” anode and cathode are returned to their original “charged” state. Much of the engineering involved in the design of rechargeable batteries involves the management of gasses generated during cycling — careful control of charge voltages and currents are critical in this management scheme, as is consideration of gas recombination mechanisms, where appropriate. Properly designed, a secondary battery can be recharged hundreds of times (with shallow cycling, many thousands of cycles can be achieved).
Primary Systems
The most popular primary battery used in portable consumer products is the 1.5 Volt standard alkaline battery (Zn/MnO2). Well over one billion are manufactured and sold annually. One of the alkaline battery’s many competitors are primary lithium metal batteries, but in replacing Zn/MnO2, system voltage and cost becomes a factor. Of the many different types of primary lithium battery chemistries in the marketplace, the most common is lithium/manganese dioxide (Li/MnO2). These 3.0 Volt systems are primarily used for memory backup and in consumer products such as cameras and toys. Despite their better performance, Li/MnO2 batteries have great difficulty in competing with alkaline batteries in many consumer products because of their price. Upcoming in popularity is the 1.5 Volt lithium/iron disulfide system (Li/FeS2), which, because of its voltage, is a direct replacement for standard alkaline. On high rate applications, Li/FeS2 provides a 5X service life advantage over Zn/MnO2. On moderate to low rate applications, there is no service life advantage. Does the 5X high rate performance justify the 3X price increase? This simple example serves to highlight the performance/cost trade off offered across the board when considering various battery systems for specific applications.
Metal/air batteries are another category of primary batteries. These hold a huge volumetric energy density advantage over their competition because the active cathode is not contained within the cell — the cathode is the O2 in the air, external to the cell. As a consequence, a much larger portion of the cell’s internal volume can therefore be devoted to the anode. The metal anode occupies a far greater space than in a conventional primary battery, which results in a very high energy density. A number of different metal/air systems have been the subject of research (with Li/Air being the most coveted), but only the zinc/air battery has achieved extensive use. In the consumer market, Zn/air button cells are used almost exclusively for hearing aid applications.
Battery Market in Change
The use of lead-acid batteries (Pb/Ac) began in the nineteenth century. Because of low manufacturing costs, good performance and long life, the lead-acid battery is, in spite of its respectable age, still the most common battery in the entire world, with a market share of as much as 40 – 45 %. New manufacturing methods, cell designs and application areas are still introduced. The lead-acid battery has a wide field of applications. The most common use is as a starter battery in cars, with additional applications in industrial trucks and as reserve power. In the EV arena, Pb/Ac is well positioned to play a dominant role with micro-hybrids (start/stop hybrids)
NiCd batteries are a mature and thoroughly tested battery technology that was patented in 1899 by Waldemar Jungner. NiCd batteries are used in a huge variety of stationary, mobile and portable applications, ranging from large-scale backup power and start batteries for aircraft to handheld power tools and toys. Due to stricter EU environmental legislation, NiCd batteries are expected to gradually be phased out in Europe, at least in consumer electronics applications. However, NiCd batteries will still have a strong position on several niche markets, for many years ahead.
The NiMH battery exploits relatively new battery technology, which began to be used more at the beginning of the 1990s. NiMH batteries offer the same cell voltage as NiCd batteries, and can therefore replace them in many applications without special modifications being necessary. The cell voltage, combined with higher energy density and better environmental properties, are the driving forces that enabled NiMH batteries to win market share from NiCd during the 1990s in consumer electronic products, such as computers and mobile phones. Today, the significantly more energy-dense Li-ion batteries have completely taken over the computer and mobile phone battery markets. The portable NiMH batteries are, however, still expected to remain on the market in the near future as a low-cost alternative to lithium batteries. With their longer track record, relatively high energy density, and their good cyclic properties, NiMH batteries have also found applications in electric and hybrid vehicles.
Energy-dense Lithium-ion Batteries
Li-ion batteries were introduced onto the market in the mid 1990s and then began, as previously stated, to replace the NiMH batteries in mobile phones, notebook computers, and other portable electronic devices. At the present time, use of lithium batteries has been widely spread to other and cheaper consumer products. It is important to remember that Li-ion batteries are a generic name for a large number of different battery chemistries with varying properties and performance and therefore also suited for a wide range of products. At present, the development of lithium-ion batteries is mainly driven by the automotive industry and their need for an improved energy storage solution for their electric and hybrid vehicles.
Manufacture of lithium-ion batteries is primarily located in Japan, Korea, and China. Europe and North America are late to the game, but are coming on strong with significant investment. Li-ion batteries are still in a relatively early phase of development, seen in terms of the battery industry, and have only been readily available for 15 years in the commercial market. This means that there is a potential for both comprehensive technical development and price reductions.
Selecting the Most Suitable Battery for Your Application
The choice of primary or secondary batteries depends on financial factors and the technical requirements imposed by its intended use. In general, primary batteries are principally used for applications with low energy consumption, in which there is a long storage time between use, or where it is difficult or inconvenient to charge the battery. Secondary batteries are primarily used when there is a need for high levels of energy or large load currents, and where it is convenient to charge the batteries. Although rechargeable batteries have a greater initial investment cost, they are cost effective in high energy applications where one rechargeable battery can be take the place of many primary battery replacements. It is interesting to note that on single use applications, primary batteries hold a 10% to 50% advantage in energy density. Design for rechargeability and long cycle life requires compromises in material use and construction. Cost is also a factor.
There is a wide range of primary and secondary batteries. The most common primary systems are alkaline, lithium metal and zinc/air batteries. Among secondary batteries, lead acid, nickel/cadmium (NiCd), nickel/metal hybrid (NiMH) and Lithium-ion (Li-ion)/Lithium-polymer (Li-polymer) batteries dominate. Ongoing battery research is constantly striving to develop new systems that can match or exceed the performance of existing systems, improve their safety, and reduce their cost.
Your Lithium Battery Options
The expression “lithium batteries” is a generic name for battery technologies in which lithium ions play a part in the primary electrochemical discharge and charge reactions. Lithium batteries are available both as primary batteries (disposable batteries) and secondary batteries (rechargeable batteries). The choice of the best battery for a specific purpose is determined by a number of parameters, such as the power and energy requirements of the application, the operating environment, the electrical preconditions and the cost aspects. The most important factors are:
- The type of battery – disposable or rechargeable battery
- Electrochemical system – matching the benefits and disadvantages of the battery type to the requirements of the application.
- The voltage requirements – rated and operating voltage respectively, the minimum and maximum levels, voltage regulation, discharge profile, etc.
- Load profile – type of load: resistive, current or power discharge, single vs. variable load, etc.
- Work cycle – continual or intermittent
- Temperature specifications
- Lifetime
- Physical requirements – size, shape, connections, etc.
- Storage requirements – shelf life, charge state when stored, environment, etc.
- Charge and discharge states (rechargeable batteries) – cycling batteries vs. energy storage batteries (flow charging), charge characteristics, efficiency and accessibility, discharge requirements
- Environmental conditions – climate (temperature, humidity), pressure conditions, physical environment (impact, vibration, dust, acceleration, etc)
- Safety and reliability – permitted variance, acceptable faults, sensitivity to gas leaks or leaks of electrolyte from the battery, etc.
- Unusual or demanding operating conditions – e.g. extreme temperature conditions, standby times, high reliability and rapid activation, etc.
- Maintenance and replacement of unserviceable batteries
- Costs – initial, and lifetime operating costs
Note that although cost is at the bottom of this list, for many applications it is the number one consideration.
Lithium-based battery systems are characterised by high energy density levels, relatively high voltages, and low weight-to-volume ratio but, in general, tend to be more expensive than equivalent battery technologies with aqueous electrolytes, such as alkali disposable batteries and zinc/air batteries in the primary battery sector, and nickel metal hydride, nickel cadmium and lead batteries in the secondary battery sector. Because they are aqueous based, alkaline batteries are also viewed as being more safe than flammable organic electrolyte containing lithium batteries. This is NOT to say that lithium batteries are not safe..
Primary Lithium Batteries
Primary lithium batteries have existed since the 1970s, are easy to use and provide convenient sources of energy for portable applications. The batteries usually require no or
very little maintenance and they have a long shelf life; modern lithium batteries can usually be stored for up to 10 years, and there are special batteries with solid state electrolyte that can be stored for more than 20 years. The storage tolerance at elevated temperatures is generally good, in some cases up to 70°C.
There are 3 classes of primary lithium batteries:
- Solid state batteries – Characterized by low power but superior shelf lives, and are used, for example, in pacemakers and computer memory backup. The expression ‘solid state’ is used because the electrolyte is solid and the ion transmission between the electrodes takes place in a solid, non-electrically conductive material, usually a polymer.
- Batteries with a solid cathode – Usually found in coin cells or small cylindrical batteries and used in a huge numbers of applications, such as clocks, memory circuits, photo and communication equipment, toys, flashlights, etc.
- Batteries with soluble cathodes – Industrial and military batteries that are manufactured in large cell sizes, up to 35-40 Ah, but are also available in smaller sizes, used, for example, in medical and civilian applications.
All of these batteries have lithium metal or lithium alloy anodes (the battery’s negative terminal). The cathode material determines the battery system.
The two most common primary lithium batteries on the market are lithium disulphide (LiFeS2) and lithium manganese dioxide (LiMnO2) batteries. Both of these are of the solid cathode type and are sold as consumer batteries from electrical goods stores and supermarkets. Other primary lithium batteries are mainly intended for the professional market. Table 1 lists the most common primary lithium battery chemistries and their properties.
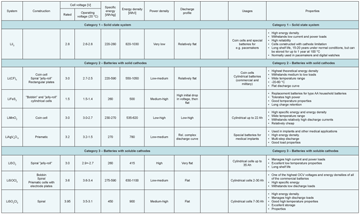
Table 1: Primary lithium batteries Click for full view
Over the last few years the market has seen the introduction of battery types in which the cathode consists of a mixture of different electrode materials. The purpose of these batteries is to provide a system that can accommodate both high and low loads. One example is the battery that is used for implanted defibrillators that consist of silver-vanadium oxide (SVO) cathodes to absorbs the peak load of a defibrillation, and carbon monofluoride ((CF)x) for the baseline load between the defibrillation pulses.
Secondary Lithium Batteries
Today, nearly all rechargeable lithium batteries are of the lithium ion type, having a negative electrode that consists of a carbon-based material, usually graphite, or another type of alloy or material that permits intercalation, i.e. storage, of lithium in the structure. This category includes lithium polymer batteries, which differ from traditional lithium ion batteries in that they have an electrolyte that is bound within a non-conducting polymer matrix. Lithium ion batteries were introduced by SONY in 1991, and were first used in advanced consumer electronics products, mobile phones, digital still and video cameras, as well as laptop computers. This type of battery is constantly gaining new ground, and the areas of use are constantly expanding, and lithium ion batteries are currently used in all sorts of portable applications within consumer electronics, medical technology and military systems. The general properties that contribute to this are the high energy density of the lithium ion battery and its specific energy, i.e. a lot of energy from a small volume and weight, compared with other rechargeable types of battery. Its other properties include low self-discharge, relatively long recharge lifetimes (300-500 complete charges and even more if the battery is used within a more restricted charge state range). High costs and the safety aspects are the main barriers. Existing lithium ion batteries suffer from functional limitations at low temperatures and degrade rapidly at temperatures exceeding 55C.
Carbon materials are used in lithium ion batteries because carbon has the ability to reversibly absorb and release large quantities of lithium (Li:C=1:6) without altering the mechanical and electrical properties of the material. Graphite and coke also have an electrode potential that is close to that of lithium, 0.0-0.1 for graphite, and 0.0-0.3 for coke. On the first charge of the battery (i.e. transport of lithium into the carbon), the a protecting outer layer is formed (Solid Electrolyte Interface, SEI). The SEI layer is important because it prevents the carbon anode from reacting with the electrolyte. Dissolution of the SEI layer at elevated temperature poses an important safety risk to lithium ion batteries.
There are a number of different chemistries within the lithium ion family that are based on the choice of chemistry in the cathode material. Traditional lithium ion batteries use cathodes made from cobalt dioxide (LiCoO2). By adding nickel (LiNi1-xCoxO2), a layered material is obtained that is more stable and less expensive than pure CoO2. Manganese oxide spinel (LiMn2O4) is another common cathode material for lithium ion batteries. Compared to cobalt, manganese oxide is cheaper but has a lower energy density and is less stable than cobalt in terms of calendar and cycle life (note that from a thermal/safety perspective, cobalt is generally considered the least stable cathode chemistry — but among the highest in terms of energy density). Extensive research is being carried out into new cathode materials, and in the last few years lithium ion batteries with cathodes made from lithium iron phosphate (LiFePO4) have been marketed on a large scale, based on their higher safety levels and low cost compared with conventional lithium ion systems. The benefits of LiFePO4 have been achieved at the expense of their energy density and voltage. New electrode materials are being developed and tested constantly in order to produce lithium ion batteries with properties that are suitable for different applications and areas of use. Table 2 shows a comparison of the main types of lithium ion batteries that are currently on the market. All of them have graphite as the most commonly occurring anode, but there are also high power batteries using hard carbon. At present, research into rechargeable lithium ion batteries is being driven mainly by the requirement in the automotive industry for long calendar and charge lifetimes, high discharge currents and the need for rapid charging, in addition to cost targets.
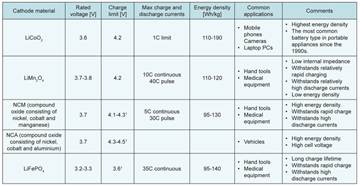
1 Charging at higher voltage leads to fewer charge cycles
Trends in Lithium Ion Batteries
Trends that are noticeable within research & development of lithium ion batteries are:
- A transition to cheaper and less toxic electrode materials (cathodes), e.g. phosphates and silicates.
- The transition to materials that have higher reversible lithium reception. The more lithium atoms that the material can absorb in each unit cell, the higher will be the potential battery capacity.
- Materials that can withstand rapid charges (from 0 to 90% SOC in ten minutes)
- Power and energy batteries for the automotive industry and stationary installations.
- Increased cell size in the form of storedenergy capacity.
- Battery systems with high voltage levels, including electrolytes that can withstand higher electrode potential without degrading or reacting with the environment.
- Battery systems with enhanced safety compared to current battery types.
Titanate, silicon and silicides, as well as tin and tin alloys, are new anode materials that have been discussed in the context of research and development. All of these materials are regarded as offering improved safety as they do not form an SEI layer. Recently, titanate as well as mixed anodes of tin, carbon and silicon have started to enter the commercial battery market. The main advantage of titanate is that its structure is highly stable and there is no increase in volume during battery charging. Lithium’s mobility within the material is very rapid, which permits the battery to be charged and discharged at high currents. The limitations include low cell voltage, due to the high electrode potential of titanate compared to that of lithium intercalation into carbon. In addition, the electrodes are also more expensive than carbon-based electrodes. Silicon and tin are limited both by very large electrode expansion during charging, around 300 and 250% respectively. However, both of these material groups are attractive because of their high energy density as well as relatively low electrode potential. Silicon is especially interesting as it permits storage of large quantities of lithium in the anode structure, e.g. the reversible Li ion insertion capacity is >1. Moreover, the material is neither expensive nor toxic.
Development on the cathode side is more multi-faceted. The list of substances and variants of substances that are being studied is extremely long, and many of them will never be marketed. Some of them will only be of interest for niche applications on cost grounds. Among the materials being discussed are metal phosphates (in particular vanadium, magnesium and manganese, as well as various compounds), layered metal oxides (often with manganese oxide bases) and silicates (e.g. iron silicate). The metal phosphates are being promoted thanks to their chemical stability, leading to increased safety compared to traditional cobalt-based cathode materials. Magnesium and manganese phosphates are also relatively cheap and non-toxic materials, which is also one of the strengths of iron silicate. The layered metal oxide structures are expected to offer rapid lithium mobility, thereby permitting high charge and discharge currents, but the material is still relatively expensive and associated with safety problems.
In summary, lithium batteries are expected, over the next 20 years, to dominate the market for rechargeable batteries, while NiCd and NiMH batteries will still be used in some niche applications.
Risks/Safety Factors for Lithium Batteries
While one of the lithium battery’s major strengths is its high energy density, high energy content is responsible for lithium-based chemistry’s down side – safety risk, when compared to other battery systems. Although the risks are directly linked to the specific cell chemistry, cell size and the number of cells in the battery, there are certain common factors. Lithium batteries contain flammable material in the form of organic electrolytes that have a low flash point and polymers that can maintain a fire and increase the risk of spreading to surrounding areas. The anode in primary lithium batteries consists of metallic lithium that melts at 180°C (when molten, lithium metal is much more reactive and mobile, creating extensive internal short circuits across and around the separator system). Both lithium metal and charged Li-Ion anodes react violently with water, generating highly flammable (explosive) hydrogen gas. The cells also contain several compounds that are toxic themselves and which form harmful combustion products in the case of fire.
One should always try to avoid exposing batteries to heat. This is partly because the cell chemistry may become unstable if the temperature is too high, which can lead to venting, and in the worst case scenario, to explosion and fire. Elevated temperatures accelerate the normal chemical processes taking place in the battery cell, leading to cell aging and loss of capacity. Heat can either be generated by the cell itself (decomposition, internal short circuits and also during normal usage) or come from an external source. Uneven heat across the cells in a battery pack is an insidious problem in that the temperature differential will cause the cells to age at different rates. With time, this will lead to problems in cell balancing. Cell balancing relates to how cells perform together inside a battery pack. The greater the spread in max/min cell capacities, the quicker a battery pack will lose capacity and fail. This is why, during battery pack assembly, much care is taken to choose cells whose capacity and impedance are well matched. A battery stack is never stronger than the weakest cell.
Low temperatures can also pose a problem. Primary batteries generally only lose their function when it becomes too cold. Rechargeable batteries often have limited chargeability at low temperatures, and should not be charged if the ambient temperature is lower than the lowest recommended charge temperature, as it may lead to formation of metallic lithium (aka lithium plating). Lithium is well known to form dendrites when plated and these can result in internal cell short circuits if the dendrites pierce the separator
barrier membrane.
Common External Causes of Battery Faults
Some of the most common causes of battery faults originating from the user or application include:
- External short circuit of the battery
- Too high discharge or charge current
- Pole reversal, i.e. discharge of a cell below 0V
- Charging primary batteries
- Incorrect or insufficient charge control (this is one key role of the BMS, the battery management system).
All five of these conditions generate heat to a varying extent in the battery cell, which leads to increased aging or breakdown of the cell. As these risks are caused by external factors, it is possible to prevent and avoid them.
External short circuit
When battery poles are short circuited, the high short circuit current leads to generation of a lot of heat in the cell. The most efficient way of preventing this from happening is to employ smart battery design that ensures that the + and – poles are physically isolated from each other, by countersinking them into the battery casing and designing them in such a way that it is not possible to connect the battery incorrectly. In certain cases, it may be necessary to integrate further forms of protection in the form of a fuse, or a PTC (Positive Temperature Coefficient) component, which breaks the electrical circuit if the current or the temperature begins to run away. Certain cell manufacturers integrate PTCs in their cells. PTCs are commonly found integrated into the caps of cylindrical sized cells, such as the 18650.
It is also important to be aware of the risk of a short circuit when transporting individual cells or batteries. The rules that exist for packaging lithium cells and batteries, with or without equipment, are intended to prevent accidents from accidental short circuits.
Too high discharge or charge current
If the current is too high, the mass transport of the reactants for the main reactions is limiting, and the energy supplied is partially consumed by side reactions in the cell, such as gassing and decomposition, which lead to increases in temperature. If the temperature increase is too high, it leads to further side reactions, which in turn generate even more heat. This increases the risk of venting and, in the worst-case scenario, uncontrolled cell reactions and thermal runaway.
When charging secondary cells, it is important not to exceed the recommended maximum charge current. If the charge current is too high, there is a risk of the lithium ions not managing to diffuse into the anode structure during charge, and instead being precipitated as lithium metal on the anode surface. This not only results in loss of cell capacity but also increases the risk of internal short circuits during subsequent use.
Apart from current limiters, it is important that there is a low voltage protection to prevent any cell discharging below the lowest recommended voltage limit of the cell. In rechargeable batteries, the low voltage protection prevents the anode’s base metal, usually copper, from dissolving and contaminating the cell. Li-ion cells that are heavily discharged risk both irreversible loss of capacity and increased self-discharge in subsequent use. The dissolved copper may also serve as starting point for creating an internal short. Rechargeable batteries also need to be fitted with an over-voltage protection to prevent early aging. Depending on the application and the complexity of the battery, it is not always sufficient with over-voltage and low voltage protection at the stack level, and control may have to be implemented on an individual cell level within the battery stack.
Pole reversal
Pole reversal can take place in battery stacks that consist of multiple cells connected in series. It is important to make sure that the cells in a multi-cell battery stack are well matched to begin with, and they are exposed to mainly the same loads and other conditions, so that the capacity continues to be evenly distributed. Otherwise, in the worst case scenario, the weakest cell could risk being heavily discharged and forced to reverse its poles, which means that the cell would be charged by the other cells, which are still being discharged. Multi-cell battery stacks usually have a cell-balancing function that distributes the current between the cells in a suitable manner. If the battery stack is fitted with low voltage protection, this may help to prevent pole reversal. The pole reversal will lead to a number of unwanted reactions within the battery which leads to heat generation which has consequences both on aging and safety.
Charging primary batteries
Primary lithium batteries are not constructed to be charged. If this takes place, gas is formed within the cell, causing its internal pressure to rise. This may result in venting, or most seriously, in explosion. Primary lithium cells connected in parallel, and thereby exposed to a potential source of charging, should be protected by diodes.
Incorrect or insufficient charge control of rechargeable batteries
Charging is the single most risky element in the battery cycle, as energy is permitted to flow into the system from an external source. It is extremely important to comply with the charge recommendations of the cell and battery manufacturers, both with respect to current limitation, voltage limitation and temperature, in order to ensure a long and safe battery life. The risks involved in too high charge current, exceeding the voltage limits, and too high and low temperatures, have been discussed above.
Internal Causes of Battery Failure
In addition to external fault sources, there are risks that may be difficult to predict or prevent, and which are caused by unsuitable cell design, manufacturing faults or ageing mechanisms within the battery cell, such as:
- Weak or faulty seals that can cause leakage or failure
- Contaminants that can lead to gas-developing secondary reactions, resulting in aging or venting due to overpressure in the cell
- Internal short circuits caused by formation of dendrites on the electrodes, metal particles or faulty insulation of conductors, etc.
Primary lithium batteries
Primary lithium batteries have a higher energy density than the rechargeable Li-ion and Li-polymer cells. Small primary cells, e.g. coin cells, often lack the integrated safety components at cell level, but are considered safe simply because of their small energy content. Larger cylinder batteries and prismatic cells often have so-called safety valves, which is a form of a pressure vent, fuse, or PTC.
The greatest safety risks are involved when a battery consists of several cells. In such cases, the cells should be “permanently connected” together in a battery stack to lower the risk of the user mixing different types of cell, and cell chemistries when changing batteries. Even if the battery cells have integrated overheating protection, it is recommended that the battery is fitted with an external temperature limitation protector. The electrical protection must also include protection against charging, short circuit and a bypass connection protection that prevents pole reversal of an individual cell in a series, or a combination of serial and parallel connected cells.
Rechargeable lithium ion batteries
Most of us have seen images and videos of burning laptops on the Internet, TV and in newspapers. For those not deliberately induced by overcharging or heating, analysis of these “field failures” show that these were usually caused by an internal short circuit arising from debris during manufacture. The short circuit leads to the cell’s energy being discharged through a very limited volume, which leads to a rapid local temperature increase, reaching up to > 200°C in seconds. The heat induces the electrodes and electrolyte to begin to decompose, and these reactions add further heat, tipping the cell into so-called thermal runaway in which the cell temperature and pressure increase exponentially. The overpressure leads to the cell venting, which liberates electrolyte aerosol in the surrounding area, and can easily ignite. In extreme cases, the cell splits or explodes unless the overpressure can be ventilated out sufficiently quickly. Depending on the construction of the cell and the internal positioning of the cells in a battery pack, the ignited electrolyte from one cell may heat up the adjacent cells and causes the cascade effect. This is particularly true for a series stack of cylindrical cells whose venting electrolyte ignites and behaves like a torch, with focus, directional burning of pressurized flammable organic electrolyte solvents.
Most Li-ion batteries on the market today indicate 60°C as their upper usage temperature. The majority of batteries currently on the market have graphite anodes. Graphite is not stable and can react chemically with the electrolyte in the cell. During manufacture and formation of the cell, a passive layer is formed on the anode surface, the SEI (Solid Electrolyte Interface) layer, which prevents continued reaction between the electrode and the electrolyte. Already at temperatures below 90°C, the layer can begin to break down, allowing exothermal gas evolving reactions to begin. If a sufficiently large area of the SEI layer breaks down, the cell may enter thermal runaway.
Not all cells that develop an internal short circuit suffer thermal runaway. Factors that influence this include:
- The size and location of the short circuit
- The size and design of the cell
- The active material in the cell; cobalt oxide is, e.g. more reactive than manganese dioxide and iron phosphate
- Thermal properties of the various materials in the cells and the cells’ abilities to disseminate heat
- The cell’s age and history and perhaps most importantly, the cell’s state of charge.
Internal short circuits caused by production factors may occur in all types of Li-ion cells and it is unlikely that it will be possible to completely eliminate this type of fault. There are various types of integrated protection at cell level that attempt to prevent and counteract risks.
Figure 1 shows a cut-through of a cylinder cell. At the top of the cell there are 2 mechanical protectors in the form of a CID (current interrupt device) that triggers at a pressure of around 10 bar, and a safety valve. Both of these are irreversible, and the cell no longer works if either of these protectors is triggered. In addition, there is usually a PTC that throttles the current at temperatures over 125°C.
Figure 1: Cut-through of a Li-Ion cylinder cell
This PTC function is reversible and conduction restarts when the temperature falls. The separator between the anode and the cathode typically consists of 3 layers of polymer and is usually of the “shut-down” type, which means that when the cell temperature gets too high, around 165°C, the separator melts and the pores close. The consequence is that the electric circuit is broken as the ion conduction in the cell ceases to function. This effect is irreversible. A number of additives have also been added to the electrolyte in the form of flame retardants or inhibitors and redox shuttles. The role of the redox shuttle is to manage and consume any overcharge effect so that the cell voltage does not get too high. The cell container is also a part of the total protection which secures the cell’s integrity against its surroundings.
The increasingly more common “pouch” cells, i.e. cells with a soft coating that consists of a polymer-coasted metal foil, lack many of the mechanical protection components that are found in cylindrical and prismatic cells with steel or aluminum casing. Pouch cells have no integral CID, PTC or safety valve, but are completely dependent on external protection circuits. In the case of a strong overpressure, the cell’s welded seams split, generally in vicinity to the pole connectors where the weld is the weakest. Nor are pouch cells as resistant to impact or puncturing as cells with metal casing.
Testing & Evaluating Lithium Batteries
In order to ensure that a battery-driven product functions in a good way, there are different methods of testing and evaluating the battery’s functioning and safety. There are four different categories of tests that can be used in order to qualify a battery:
- Electrical performance testing – different types of test that investigate the battery’s electrical functioning, for example available capacity at different loads and surrounding temperatures, cycle lifetime, calendar lifetime in storage, charging receptivity, impedance as a function of charging state etc.
- Safety testing in relation to environmental effects – various tests that simulate different kinds of environmental conditions to which the battery could be exposed, for example, vibrations, falls, knocks and blows, damp, high temperatures or quick temperature changes.
- Safety testing in relation to faults or incorrect usage – various tests that evaluate the battery’s ability to deal with different types of stress that can arise intentionally or unintentionally when using the product, for example, overcharging, short circuiting, incorrect installation and similar situations.
- Effect on the environment – chemical analysis of heavy metal content.
Some of these tests have to be carried out because of rules and legal requirements. Amongst these is transport testing in accordance with the UN’s transport rules for dangerous goods (UN Manual of Tests and Criteria section 38.3) which is required in order to transport lithium batteries and cells and products that contain lithium batteries, irrespective of the type of transport. Several countries apply limits for certain heavy metals in batteries. The chemical elements that are most commonly regulated are mercury, cadmium and lead. The EU Battery Directive regulates all three of these elements.
Electrical Performance Testing
A battery’s technical specifications say a lot, but not everything, that an instrument manufacturer needs to know about the battery cell or battery pack that has been chosen to power a certain apparatus. Available battery capacity and the number of discharge cycles are two factors that are strongly affected by actual conditions of use. Similar battery cells from different manufacturers and even different models from the same manufacturer do not need to have the same properties, since the functioning of the battery is governed by those chemical reactions that are possible in each individual case. The balance between different components, additives, pollutant contents etc. are very important to cell chemistry. Cell design and the manufacturers’ recipes for electrode and electrolyte composition are carefully guarded secrets and are important competitive tools among manufacturers. The extent of the testing which is carried out by cell and battery manufacturers can also vary from case to case. Testing represents a cost, and one can therefore assume that low-budget products in many cases have undergone less extensive testing than advanced products from more renowned and experienced manufacturers.
Minimum Requirements in Standards
The type tests that are found in IEC standards are basic tools for evaluating lithium cells and batteries’ electrical properties. IEC 60086-1 and IEC 60086-2 lay down dimensional requirements for different cell types and sizes of primary batteries. The corresponding standard for rechargeable cells and batteries is IEC 61960. It is generally the case that standard requirements are minimum requirements that all batteries must comply with. Most modern, battery-driven products available on the market impose higher or more extensive requirements on their batteries and so standard tests should be supplemented with application-specific discharge and lifetime tests.
When buying cells and batteries, one should demand that they fulfill the relevant standard requirements. Cell and battery suppliers should be able to provide reports from tests carried out and be able to account for the extent and frequency of tests, both as part of ongoing production checks and those that are carried out in final checks on the finished cells and batteries. It is not unusual for low-budget cell and battery specifications to state that the product is designed to conform to the requirements of IEC standards without any such testing having been carried out, or that limited testing is carried out covering only parts of the standards. In some cases testing has been performed in connection with the original product launch but is not updated in connection with product development or changes in components.
Safety Testing for External Influences
The UN’s transport testing is a typical example of safety testing designed to establish the battery’s safety properties in the event of external influences. The test program includes a total of eight tests designed to simulate conditions that could occur in a transport situation.
- T1 Simulation of high height (test to recreate low pressure when flying)
- T2 Thermal shock (exposing the battery to alternating high and low temperatures)
- T3 Vibration test
- T4 Fall impact test
- T5 External short-circuiting
- T6 Blow test
- T7 Overcharging test
- T8 Forced discharging
It is important to note that it is not enough to test the individual cells that are included in a battery pack and that the whole battery pack must be tested if it consists of several cells. Re-testing is also required if the product is modified in a way that could affect the results of testing and if the product’s weight (primary lithium batteries) or Wh-content or voltage (rechargeable lithium cells and batteries) are changed. As well as special testing, transport rules require that lithium batteries and products that contain lithium batteries are packed, marked and accompanied by safety documentation in accordance with given requirements. Some countries/transport authorities set higher requirements than those that normally apply in UN 38.3. Today, transports in the USA are subject to more extensive requirements.
UL and IEC standards consist of a combination of environmental influence tests and tests that simulate predictable types of incorrect use. The tests focus on evaluating fire-safety and the requirement for approval is generally that the cell or battery does not explode or burn during the course of a test. The tests included in UL or IEC are similar, even though there can be differences in the degree of strictness between the different standards. UL holds a strong position in North America whilst IEC dominates in the rest of the world.
When buying cells or batteries, you should insist that the cell or battery supplier has carried out tests in accordance with the UN’s transport requirements. If these have not been carried out, this can affect the timetable for the product launch and involve considerable costs for the equipment manufacturer, who must then take responsibility for carrying out the tests. Cell and battery suppliers should also be able to provide test reports from completed testing in accordance with UL 1642 (cells) and 2054 (battery packs) or IEC 60086-4 (primary lithium cells and batteries) or IEC 62133 (rechargeable cells and batteries) and to account for the extent and frequency of tests carried out, both as part of ongoing production checks and as final checks on the finished cells and batteries. If, as a buyer, you are unsure whether the battery has been tested or whether the testing has been carried out in the right way, then you should carry out your own verification in accordance with relevant standard methods.
Regulated Heavy Metals are Uncommon
There is no named method that has to be used for chemical analysis of batteries. None of the listed substances; mercury, cadmium or lead contributes to the electro-chemistry in lithium batteries. There is therefore no reason for manufacturers to add them intentionally. To the extent that they do occur, it’s in the form of raw material contamination. It is extremely unusual for lithium-based cells and batteries to contain problematically high levels of these substances.
Battery Testing Standards
Below is a list of the testing standards mentioned earlier. Most national and regional standards, such as SS and EN, are based on the corresponding IEC standard and this is therefore referred to in the current version of IEC. In some cases, national standards are not updated at the same time as IEC and for that reason it is important to be certain that testing has been carried out in accordance with the current guidelines and, where necessary, supplement the tests carried out with further tests.
[Note: ANSI C18 series essentially contains the US equivalent of IEC battery standards]
For transport rules, the method of transport governs which guidelines that apply, i.e. ADR/RID for land transport, the IMDG-code for sea transport and the ICAO-TI and IATA-DGR for air transport. These are updated regularly, but there is sometimes a time delay which leads to transport law guidelines referring to an earlier edition of the UN Manual of Test and Criteria.
UN’s transport testing for all batteries that contain lithium:
ST/SG/AC.10/11/Rev.5; UN
Manual of Tests and Criteria, Rev.5 (2009)
Primary batteries:
- IEC 60086-1: Primary batteries – Part 1: General,Ed 10.0 (2006)
- IEC 60086-2: Primary batteries – Part 2: Physical and electrical specifications, Ed. 11.0 (2006)
- IEC 60086-4: Primary batteries – Part 4: Safety of lithium batteries, Ed. 3.0 (2007)
- UL 1642: Lithium Batteries, Ed. 4, (2005, withrevisions 11/2009)
- UL 2054: Household and Commercial batteries (2004, with revisions 11/2009)
Rechargeable batteries:
- IEC 62133: Secondary cells and batteries containing alkaline or other non-acid electrolytes – Safety requirements for portable sealed secondary cells, and for batteries made from them, for use in portable applications, Ed. 1.0 (2002)
- IEC 61960: Secondary cells and batteries containing alkaline or other non-acid electrolytes – Secondary lithium cells and batteries for portable applications, Ed.1.0, (2003)
- UL 1642: Lithium Batteries, Ed. 4, (2005, withrevisions 11/2009)
- UL 2054: Household and Commercial batteries (2004, with revisions 11/2009)
Note that several of the listed standards are currently in revision.
Construction Requirements for Battery Powered Equipment
When applying for product certification for medical-technical equipment, an approved result of the safety test in accordance with IEC or UL standards constitutes a basis for being able to approve the battery as a safe component. This means that if there are several suppliers of cells and/or batteries for a specific item of equipment, then they must be approved individually in order to be used in the equipment.
CB-certification of products containing rechargeable Li-ion batteries requires testing according to IEC 62133 as of May 1, 2012, and that Li cells and batteries certified to UL 1642 and UL 2054, respectively, have to undergo and fulfill additional testing in order to conform to IEC 62133. Primary lithium batteries must be certified in accordance with IEC 60086-4. The corresponding timetable for products falling under IEC 60065 and IEC 60950-1 remains to be determined.
Product certification requires that the equipment must be safe if a component fails. Fire or dangerous explosions must not occur and a risk analysis is performed based on the test results of the device. For a primary battery or cell of the lithium type, protection is only required against reverse current. It may also be appropriate to specify regular checks on the protective components. For batteries or cells that are tested and certified as short-circuit-proof, no further protection is needed. For other batteries a current-limiting component is also required to limit the discharge current. The reverse and discharge currents that the battery is certified for must not be exceeded in the case of a fault.
For secondary lithium batteries, i.e. rechargeable batteries and cells, the charging battery protection circuits are investigated. The battery must be protected against excessive discharge current, charging current and charging voltage. Where appropriate, it may also be necessary to monitor the battery’s temperature in order to shut down the device and mitigate the effects in case of over-temperature. If the battery protection is not certified, two independent protective measures are required for each of the three parameters, which is the most commonly occurring scenario.
In addition, a risk analysis based on testing should be carried out to show that it is improbable that faults should occur in both protective circuits simultaneously.
Environmental Considerations for Lithium Batteries
There is a growing awareness of interest in environmental issues across all sectors of society in recent years. Many people argue that our long-term survival depends upon reducing our impact on nature and that we must stop releasing toxic materials into the environment. Batteries are not yet covered by legislation on chemicals, but the “Regulation on Batteries,” which is based on the EU Battery Directive, governs the use of certain heavy metals in batteries. Unfortunately, this legislation has not kept pace with technological developments in the battery field. This is also the case when it comes to environmental labeling requirements on batteries, such as the Swan Label.
There are at least three key factors that can be used when determining how environment friendly a battery is:
- Battery lifespan and the number of cells required to achieve the desired battery function in the equipment or apparatus
- Recyclability
- Chemical content
Upper Limits and Labeling Requirements
The EU Battery Directive is currently the most far-reaching Directive on the regulation of hazardous elements that are used in batteries. The Directive includes both fixed upper limits by weight for how much cadmium (20 ppm) and mercury (5 ppm) batteries can contain, with the exception of military and certain industrial batteries, plus batteries for emergency and alarm systems, cordless power tools and a number of medical equipment products. The Battery Directive and all related national legislation within the EU also covers labeling requirements for batteries with mercury (5 ppm), cadmium (20 ppm) and lead (40 ppm) content along with requirements on the collection and treatment of spent batteries, irrespective of the particular type of battery.
Several countries in Asia (e.g. China, Japan and Singapore) have introduced regulation on heavy metals and batteries. Parts of the USA and Canada have also come a long way on the collection and treatment of batteries.
No Mercury, Cadmium or Lead
None of the listed elements (mercury, cadmium and lead) play a part in electrochemical cells in lithium batteries and therefore, there is no reason for manufacturers to deliberately include them. Where they are found, it is in the form of contamination of raw materials used. It is extremely unusual for lithium-based cells or batteries to contain problematically high levels of these elements. This has persuaded some manufacturers of these batteries to claim their products are “green” and environment friendly. The basis for such claims is debatable as lithium batteries, and rechargeable lithium-ion batteries in particular, are extremely complex and can contain a large number of different elements in varying degrees. This relationship is also reflected in the environment labeling requirements specified by Swan, which currently regulates these three heavy metals and, in the case of rechargeable batteries, arsenic.
Rechargeable Batteries are Preferable
Generally speaking, rechargeable batteries are more environment friendly than single-use batteries when used in the same application. This is because the total amount of battery waste will be lower as the same battery can be recharged numerous times, hundreds of times as a rule. Within the group of primary batteries (single use batteries), lithium cells offer an advantage in the form of higher energy density compared to alkaline batteries, which enable a longer operating time. Most primary lithium cells also have a higher cell voltage which also means they need fewer cells to achieve the desired operating voltage in the apparatus. Both these characteristics help make primary lithium cells appear more advantageous than other primary cells from an environment perspective, as fewer cells are required to achieve the same performance and lifespan. These same arguments can be used in favor of lithium-ion cells, as these have a higher cell voltage than other rechargeable cell types.
Nickel from the Toyota Prius Can be Recycled
Recyclability is totally dependent on the availability of efficient collection systems that ensure batteries do not end up in landfill sites and that there are financial incentives to recover the materials found in batteries. Here, traditional chemical batteries (e.g. lead, nickel cadmium and nickel metal hydride batteries) are actually an advantage compared to lithium-ion batteries, as traditional batteries have a high content of metals that have a second-hand value on the commodities markets. Exhausted lead batteries can be used directly in the manufacture of new lead batteries. Nickel from nickel cadmium and nickel metal hydride batteries is used by the steel industry in the manufacture of stainless steel. However, recycled nickel is not yet of sufficiently high quality to be used in new batteries.
Toyota has just announced that they have developed a method that enables them to recover nickel from old Prius batteries that can be used in new ones. It will be interesting to follow this development and whether this method can be used for nickel metal hydride batteries of the consumer type. Cadmium can also be recovered and recycled in the production of new nickel cadmium batteries.
Spent Cell Content Used in Construction Industry
Lithium-ion batteries contain relatively small quantities of elements that are financially viable to recover. The large variety of cell chemistries available on the market also makes recycling more difficult. There are recycling processes currently available for lithium-ion batteries that recover cobalt, nickel and copper from battery waste. The residual cell content is combusted and the ash can be used in the construction industry. The trend within lithium-ion technology is moving towards a development characterized by an increased use of materials that are not of interest to recover, such as manganese dioxide, iron phosphate and mixed oxide materials with little or no cobalt in the mix. As a consequence, the cost of collection and recycling of lithium-ion batteries can largely fall on users when the manufacturers attempt to recoup their manufacturer product liabilities.
Product Life Cycle Must be Considered
The environmental impact of batteries is a very complex issue. In order to be able to evaluate and compare different batteries against each other, it is desirable to take the entire life cycle of the product into account: the extraction and refining of the raw materials, cell and battery manufacturing, product lifespan in operation plus waste disposal and recycling processes. Both manufacturers and consumers of battery-powered products should do their utmost to minimize the total number of batteries required during the lifetime of the product in order to minimize its environmental impact. It is also important to persuade users to take batteries to recycling points and to continue work on developing technology that enables as much recycled material as possible to be used.
![]()
|
Dr Maria Wesselmark, Energy Storage Specialist Dr Maria Wesselmark holds a PhD in Applied Electrochemistry from Royal Institute of Technology, Stockholm. She is working within Intertek’s advisory group as Energy Storage specialist. |
![]() |
Tom O’Hara Global Business Manager/Advisory Services, Intertek Semko, AB. Part of Intertek’s Energy Storage Business Unit covering Batteries, Fuel Cells, and Super Capacitors. A consumer battery specialist with over twenty years R&D experience with Energizer Battery. Contributions touched nearly every product in Energizer’s product portfolio. Ten years consulting with both established and start-up battery enterprises covering a wide variety of battery chemistries and applications. |